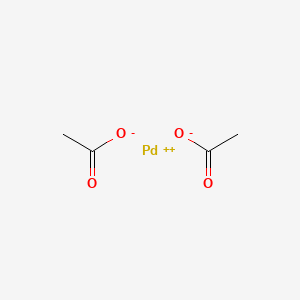
Palladium(II) acetate
Overview
Description
Palladium(II) acetate is a chemical compound with the formula [Pd(O₂CCH₃)₂]ₙ. It is a coordination complex of palladium and acetate ligands. This compound is known for its brown-yellow solid appearance and is commonly used as a catalyst in organic synthesis due to its high reactivity and solubility in organic solvents .
Mechanism of Action
Target of Action
Palladium(II) acetate primarily targets alkenic and aromatic compounds , activating them towards oxidative inter- and intramolecular nucleophilic reactions . It is more reactive than the analogous platinum compound .
Mode of Action
This compound interacts with its targets through a series of complex reactions. It is prone to reduction to Pd(0) in the presence of reagents which can undergo beta-hydride elimination such as primary and secondary alcohols as well as amines . When warmed with alcohols, or on prolonged boiling with other solvents, this compound decomposes to palladium .
Biochemical Pathways
This compound is a catalyst for many organic reactions, especially alkenes, dienes, and alkyl, aryl, and vinyl halides to form reactive adducts . It is also involved in various cross-coupling reactions . For example, it participates in the Heck reaction and related processes, rearrangement of acyclic dienes such as the Cope rearrangement, and carbonylation reactions .
Pharmacokinetics
It is known that the compound is soluble in many organic solvents , which could potentially influence its absorption, distribution, metabolism, and excretion (ADME) properties.
Result of Action
The result of this compound’s action is the formation of new compounds through various reactions. For instance, in carbonylation reactions, it can facilitate the formation of esters from aryl iodides, carbon monoxide, an alcohol, or phenol . In cross-coupling reactions, it can lead to the formation of new carbon-carbon bonds .
Action Environment
The action of this compound can be influenced by various environmental factors. For instance, the presence of certain reagents can lead to its reduction to Pd(0) . Additionally, the compound’s reactivity and stability can be affected by the solvent used, as it is soluble in many organic solvents .
Biochemical Analysis
Biochemical Properties
Palladium(II) acetate plays a significant role in biochemical reactions due to its catalytic properties. It is involved in the activation of alkenic and aromatic compounds towards oxidative inter- and intramolecular nucleophilic reactions . This compound interacts with various enzymes and proteins, facilitating the formation of carbon-carbon and carbon-heteroatom bonds. These interactions are crucial in the synthesis of complex organic molecules. The compound’s ability to coordinate with different ligands allows it to participate in a wide range of biochemical processes.
Cellular Effects
This compound has been shown to influence various cellular processes. It can induce cell death in certain types of cells, including cancer cells, by disrupting cellular metabolism and inducing oxidative stress . The compound affects cell signaling pathways, leading to changes in gene expression and cellular metabolism. This compound can also interfere with the function of mitochondria, leading to the production of reactive oxygen species and subsequent cell damage.
Molecular Mechanism
The molecular mechanism of action of this compound involves its ability to form complexes with biomolecules. This compound can bind to the active sites of enzymes, inhibiting their activity or altering their function . This binding can lead to the inhibition of key metabolic pathways and the disruption of cellular homeostasis. Additionally, this compound can induce changes in gene expression by interacting with transcription factors and other regulatory proteins.
Temporal Effects in Laboratory Settings
In laboratory settings, the effects of this compound can change over time. The compound is relatively stable under standard conditions, but it can degrade in the presence of strong acids or bases . Long-term exposure to this compound can lead to cumulative effects on cellular function, including persistent oxidative stress and mitochondrial dysfunction. These effects have been observed in both in vitro and in vivo studies.
Dosage Effects in Animal Models
The effects of this compound vary with different dosages in animal models. At low doses, the compound may have minimal impact on cellular function. At higher doses, this compound can induce toxic effects, including liver and kidney damage . Threshold effects have been observed, where a certain dosage level leads to a significant increase in adverse effects. These toxic effects are likely due to the compound’s ability to induce oxidative stress and disrupt cellular metabolism.
Metabolic Pathways
This compound is involved in several metabolic pathways, particularly those related to oxidative stress and detoxification . The compound interacts with enzymes such as glutathione peroxidase and catalase, which are involved in the detoxification of reactive oxygen species. This compound can also affect the levels of key metabolites, such as ATP and glutathione, by disrupting mitochondrial function and inducing oxidative stress.
Transport and Distribution
Within cells and tissues, this compound is transported and distributed through interactions with various transporters and binding proteins . The compound can accumulate in specific cellular compartments, such as the mitochondria, where it exerts its effects on cellular metabolism. This compound’s solubility in organic solvents allows it to be readily taken up by cells and distributed throughout the body.
Subcellular Localization
This compound is primarily localized in the mitochondria, where it affects mitochondrial function and induces oxidative stress . The compound’s localization is influenced by its ability to interact with mitochondrial proteins and membranes. This compound can also be found in other subcellular compartments, such as the cytoplasm and nucleus, where it can interact with various biomolecules and influence cellular processes.
Preparation Methods
Synthetic Routes and Reaction Conditions
Palladium(II) acetate can be synthesized through several methods:
-
Reaction with Acetic Acid and Nitric Acid: : One common method involves treating palladium sponge with a mixture of acetic acid and nitric acid. This reaction produces this compound along with water and nitrogen oxides as byproducts .
-
Reaction with Palladium Nitrate: : Another method involves reacting palladium nitrate with acetic acid. This method is advantageous as it produces a high yield of pure this compound without nitrite or nitrate impurities .
-
Reaction in Acetonitrile Solvent: : Palladium can also be reacted with acetic acid in an acetonitrile solvent, followed by crystallization or evaporation to obtain this compound .
Industrial Production Methods
Industrial production of this compound typically involves the reaction of palladium metal with acetic acid under controlled conditions. The process may include steps such as filtration, evaporation, and treatment with acetic acid ethyl ester to ensure the purity of the final product .
Chemical Reactions Analysis
Types of Reactions
Palladium(II) acetate undergoes various types of chemical reactions, including:
-
Oxidation: : It acts as an oxidation catalyst, participating in the activation of alkenic and aromatic compounds towards oxidative inter- and intramolecular nucleophilic reactions .
-
Reduction: : this compound can be reduced by light or heat to produce thin layers of palladium, which can be used to form nanowires and colloids .
-
Substitution: : It reacts with acetylacetone to produce palladium(II) acetylacetonate, a precursor to palladium(0) .
Common Reagents and Conditions
Alkenes and Aromatic Compounds: this compound is commonly used with alkenes and aromatic compounds in oxidative addition reactions.
Acetylacetone: Used in substitution reactions to form palladium(II) acetylacetonate.
Light or Heat: Applied to reduce this compound to metallic palladium.
Major Products
Palladium(0): Formed through reduction reactions.
Palladium(II) Acetylacetonate: Formed through substitution reactions with acetylacetone.
Scientific Research Applications
Palladium(II) acetate has a wide range of applications in scientific research:
-
Organic Synthesis: : It is widely used as a catalyst in cross-coupling reactions such as the Buchwald-Hartwig amination, Heck reaction, Suzuki coupling, Stille coupling, Sonogashira coupling, and Negishi coupling reactions .
-
Material Science: : Used in the preparation of palladium-containing materials for heterogeneous catalysis .
-
Biological Research:
-
Industrial Applications: : Employed in the production of supported catalysts for hydrogenation and oxidative acetoxylation of alkenes .
Comparison with Similar Compounds
Similar Compounds
Palladium(II) Chloride: Another palladium compound used in catalysis.
Platinum(II) Acetate: A similar compound with platinum instead of palladium.
Uniqueness
Palladium(II) acetate is more reactive than its platinum counterpart and is soluble in many organic solvents, making it a versatile catalyst for various organic reactions . Its unique trimeric structure and ability to form reactive palladium species contribute to its effectiveness in catalysis .
Properties
IUPAC Name |
palladium(2+);diacetate | |
---|---|---|
Source | PubChem | |
URL | https://pubchem.ncbi.nlm.nih.gov | |
Description | Data deposited in or computed by PubChem | |
InChI |
InChI=1S/2C2H4O2.Pd/c2*1-2(3)4;/h2*1H3,(H,3,4);/q;;+2/p-2 | |
Source | PubChem | |
URL | https://pubchem.ncbi.nlm.nih.gov | |
Description | Data deposited in or computed by PubChem | |
InChI Key |
YJVFFLUZDVXJQI-UHFFFAOYSA-L | |
Source | PubChem | |
URL | https://pubchem.ncbi.nlm.nih.gov | |
Description | Data deposited in or computed by PubChem | |
Canonical SMILES |
CC(=O)[O-].CC(=O)[O-].[Pd+2] | |
Source | PubChem | |
URL | https://pubchem.ncbi.nlm.nih.gov | |
Description | Data deposited in or computed by PubChem | |
Molecular Formula |
C4H6O4Pd | |
Source | PubChem | |
URL | https://pubchem.ncbi.nlm.nih.gov | |
Description | Data deposited in or computed by PubChem | |
DSSTOX Substance ID |
DTXSID10890575 | |
Record name | Diacetatopalladium | |
Source | EPA DSSTox | |
URL | https://comptox.epa.gov/dashboard/DTXSID10890575 | |
Description | DSSTox provides a high quality public chemistry resource for supporting improved predictive toxicology. | |
Molecular Weight |
224.51 g/mol | |
Source | PubChem | |
URL | https://pubchem.ncbi.nlm.nih.gov | |
Description | Data deposited in or computed by PubChem | |
Physical Description |
Reddish-brown solid; Insoluble in water; [Hawley] Powder; [MSDSonline] | |
Record name | Palladium(II) acetate | |
Source | Haz-Map, Information on Hazardous Chemicals and Occupational Diseases | |
URL | https://haz-map.com/Agents/8298 | |
Description | Haz-Map® is an occupational health database designed for health and safety professionals and for consumers seeking information about the adverse effects of workplace exposures to chemical and biological agents. | |
Explanation | Copyright (c) 2022 Haz-Map(R). All rights reserved. Unless otherwise indicated, all materials from Haz-Map are copyrighted by Haz-Map(R). No part of these materials, either text or image may be used for any purpose other than for personal use. Therefore, reproduction, modification, storage in a retrieval system or retransmission, in any form or by any means, electronic, mechanical or otherwise, for reasons other than personal use, is strictly prohibited without prior written permission. | |
CAS No. |
3375-31-3, 19807-27-3 | |
Record name | Diacetatopalladium | |
Source | ChemIDplus | |
URL | https://pubchem.ncbi.nlm.nih.gov/substance/?source=chemidplus&sourceid=0003375313 | |
Description | ChemIDplus is a free, web search system that provides access to the structure and nomenclature authority files used for the identification of chemical substances cited in National Library of Medicine (NLM) databases, including the TOXNET system. | |
Record name | Palladium diacetate | |
Source | ChemIDplus | |
URL | https://pubchem.ncbi.nlm.nih.gov/substance/?source=chemidplus&sourceid=0019807273 | |
Description | ChemIDplus is a free, web search system that provides access to the structure and nomenclature authority files used for the identification of chemical substances cited in National Library of Medicine (NLM) databases, including the TOXNET system. | |
Record name | Acetic acid, palladium salt (1:?) | |
Source | EPA Chemicals under the TSCA | |
URL | https://www.epa.gov/chemicals-under-tsca | |
Description | EPA Chemicals under the Toxic Substances Control Act (TSCA) collection contains information on chemicals and their regulations under TSCA, including non-confidential content from the TSCA Chemical Substance Inventory and Chemical Data Reporting. | |
Record name | Acetic acid, palladium(2+) salt (2:1) | |
Source | EPA Chemicals under the TSCA | |
URL | https://www.epa.gov/chemicals-under-tsca | |
Description | EPA Chemicals under the Toxic Substances Control Act (TSCA) collection contains information on chemicals and their regulations under TSCA, including non-confidential content from the TSCA Chemical Substance Inventory and Chemical Data Reporting. | |
Record name | Diacetatopalladium | |
Source | EPA DSSTox | |
URL | https://comptox.epa.gov/dashboard/DTXSID10890575 | |
Description | DSSTox provides a high quality public chemistry resource for supporting improved predictive toxicology. | |
Record name | Palladium(II) acetate | |
Source | European Chemicals Agency (ECHA) | |
URL | https://echa.europa.eu/substance-information/-/substanceinfo/100.020.151 | |
Description | The European Chemicals Agency (ECHA) is an agency of the European Union which is the driving force among regulatory authorities in implementing the EU's groundbreaking chemicals legislation for the benefit of human health and the environment as well as for innovation and competitiveness. | |
Explanation | Use of the information, documents and data from the ECHA website is subject to the terms and conditions of this Legal Notice, and subject to other binding limitations provided for under applicable law, the information, documents and data made available on the ECHA website may be reproduced, distributed and/or used, totally or in part, for non-commercial purposes provided that ECHA is acknowledged as the source: "Source: European Chemicals Agency, http://echa.europa.eu/". Such acknowledgement must be included in each copy of the material. ECHA permits and encourages organisations and individuals to create links to the ECHA website under the following cumulative conditions: Links can only be made to webpages that provide a link to the Legal Notice page. | |
Record name | Palladium acetate | |
Source | European Chemicals Agency (ECHA) | |
URL | https://echa.europa.eu/substance-information/-/substanceinfo/100.039.379 | |
Description | The European Chemicals Agency (ECHA) is an agency of the European Union which is the driving force among regulatory authorities in implementing the EU's groundbreaking chemicals legislation for the benefit of human health and the environment as well as for innovation and competitiveness. | |
Explanation | Use of the information, documents and data from the ECHA website is subject to the terms and conditions of this Legal Notice, and subject to other binding limitations provided for under applicable law, the information, documents and data made available on the ECHA website may be reproduced, distributed and/or used, totally or in part, for non-commercial purposes provided that ECHA is acknowledged as the source: "Source: European Chemicals Agency, http://echa.europa.eu/". Such acknowledgement must be included in each copy of the material. ECHA permits and encourages organisations and individuals to create links to the ECHA website under the following cumulative conditions: Links can only be made to webpages that provide a link to the Legal Notice page. | |
Record name | PALLADIUM(II) ACETATE | |
Source | FDA Global Substance Registration System (GSRS) | |
URL | https://gsrs.ncats.nih.gov/ginas/app/beta/substances/0LTG3460Y5 | |
Description | The FDA Global Substance Registration System (GSRS) enables the efficient and accurate exchange of information on what substances are in regulated products. Instead of relying on names, which vary across regulatory domains, countries, and regions, the GSRS knowledge base makes it possible for substances to be defined by standardized, scientific descriptions. | |
Explanation | Unless otherwise noted, the contents of the FDA website (www.fda.gov), both text and graphics, are not copyrighted. They are in the public domain and may be republished, reprinted and otherwise used freely by anyone without the need to obtain permission from FDA. Credit to the U.S. Food and Drug Administration as the source is appreciated but not required. | |
Retrosynthesis Analysis
AI-Powered Synthesis Planning: Our tool employs the Template_relevance Pistachio, Template_relevance Bkms_metabolic, Template_relevance Pistachio_ringbreaker, Template_relevance Reaxys, Template_relevance Reaxys_biocatalysis model, leveraging a vast database of chemical reactions to predict feasible synthetic routes.
One-Step Synthesis Focus: Specifically designed for one-step synthesis, it provides concise and direct routes for your target compounds, streamlining the synthesis process.
Accurate Predictions: Utilizing the extensive PISTACHIO, BKMS_METABOLIC, PISTACHIO_RINGBREAKER, REAXYS, REAXYS_BIOCATALYSIS database, our tool offers high-accuracy predictions, reflecting the latest in chemical research and data.
Strategy Settings
Precursor scoring | Relevance Heuristic |
---|---|
Min. plausibility | 0.01 |
Model | Template_relevance |
Template Set | Pistachio/Bkms_metabolic/Pistachio_ringbreaker/Reaxys/Reaxys_biocatalysis |
Top-N result to add to graph | 6 |
Feasible Synthetic Routes
Q1: What is the molecular formula and weight of palladium(II) acetate?
A1: The molecular formula of this compound is C4H6O4Pd and its molecular weight is 224.52 g/mol. []
Q2: What is the structure of this compound in solution?
A2: In acetic acid solution, this compound exists predominantly as a trimeric species, Pd3(OAc)6, in the absence of acetate ions. [] The addition of sodium acetate or lithium acetate to the solution leads to the formation of dimeric and monomeric species. []
Q3: How does the presence of cerium(III) salts impact this compound stability in acetic acid?
A3: Cerium(III) salts significantly enhance the stability of this compound in acetic acid. [] This stabilization is attributed to the formation of a Pd(II)-Ce(III) complex, which hinders the reduction of palladium(II) by acetic acid. []
Q4: What are some notable catalytic applications of this compound?
A4: this compound is a highly effective catalyst for a wide range of organic reactions, including:
- Transvinylation reactions: It catalyzes the transvinylation of hydroxycinnamic acids and their derivatives to produce vinyl esters. The addition of KOH or H2SO4 as co-catalysts significantly enhances the yield of these reactions. []
- Oxidative coupling of benzene: While inactive on its own, this compound, in conjunction with dialkyl sulfides, facilitates the oxidative coupling of benzene at 70°C. [, ]
- Cyanation of glycals: this compound catalyzes the cyanation of di- and trisaccharide per-O-acetylglycals with trimethylsilyl cyanide, producing di- and trisaccharidic 2-enopyranosyl cyanides in high yields. []
- Aromatic substitution of olefins: It catalyzes the arylation of various aromatic compounds, including naphthalene, ferrocene, and tricarbonyl(η-cyclobutadiene)iron(0), with styrene. [, , ]
- Carbonylation reactions: this compound catalyzes the carbonylation of isoprene in alcohol to produce 4-methyl-3-pentenoate selectively. [] It also catalyzes the methoxy carbonylation of hydroxy alkenes to synthesize functionalized tetrahydropyrans. []
- Cross-coupling reactions: In combination with tris(p-tolyl)phosphine, this compound catalyzes the highly efficient cross-coupling of benzylic halides with aryltitanium tris(isopropoxide) to yield biaryl products. []
Q5: What role does this compound play in the synthesis of benzo[b]naphtho[2,3-d]thiophene-6,11-diones?
A5: this compound mediates the oxidative cyclization of 3-arylthio-1,4-naphthoquinone, leading to the formation of benzo[b]naphtho[2,3-d]thiophene-6,11-diones, compounds with significant biological activity. []
Q6: Can this compound be used in heterogeneous catalysis?
A6: Yes, this compound microencapsulated in polyurea acts as a robust and recyclable heterogeneous catalyst for various phosphine-free cross-coupling reactions, demonstrating its utility in both conventional solvents and supercritical carbon dioxide. []
Q7: How does this compound interact with substrates during catalysis?
A7: this compound typically coordinates to substrates through its palladium center, often displacing labile ligands like acetate. This coordination activates the substrates for subsequent chemical transformations.
Q8: What influences the selectivity of this compound-catalyzed reactions?
A8: Several factors can impact reaction selectivity, including:
- Ligand environment: The choice of ligands around the palladium center can significantly influence regio- and stereoselectivity. [, , ]
- Reaction conditions: Parameters like temperature, solvent, and additives can affect the reaction pathway and product distribution. [, , ]
- Substrate structure: The steric and electronic properties of the substrates can influence the preferred reaction pathway and product formation. [, , ]
Q9: Does a hydride shift occur during the this compound-catalyzed phenylation of styrene?
A9: No, deuterium labeling studies confirmed that no hydride shift occurs during the this compound-catalyzed phenylation of styrene with benzene. [, ]
Q10: How does the alkyl group of dialkyl sulfides influence the oxidative coupling of benzene?
A10: The size and structure of the alkyl group in the dialkyl sulfide can significantly impact the rate of oxidative coupling of benzene catalyzed by the this compound-dialkyl sulfide system. []
Q11: What is the effect of oxygen atmosphere on the this compound-catalyzed oxidative coupling of benzene?
A11: The presence of oxygen is crucial for the oxidative coupling of benzene catalyzed by the this compound-dialkyl sulfide system. []
Q12: How do imidazolium-based ionic liquids impact the catalytic activity of this compound in butadiene telomerization?
A12: The use of 1,3-dialkylimidazolium salts as cosolvents can deactivate the this compound catalyst in butadiene telomerization. This is attributed to the formation of stable palladium imidazolylidene complexes. Conversely, 1,2,3-trialkylimidazolium salts offer high activity and selectivity in this reaction. []
Disclaimer and Information on In-Vitro Research Products
Please be aware that all articles and product information presented on BenchChem are intended solely for informational purposes. The products available for purchase on BenchChem are specifically designed for in-vitro studies, which are conducted outside of living organisms. In-vitro studies, derived from the Latin term "in glass," involve experiments performed in controlled laboratory settings using cells or tissues. It is important to note that these products are not categorized as medicines or drugs, and they have not received approval from the FDA for the prevention, treatment, or cure of any medical condition, ailment, or disease. We must emphasize that any form of bodily introduction of these products into humans or animals is strictly prohibited by law. It is essential to adhere to these guidelines to ensure compliance with legal and ethical standards in research and experimentation.