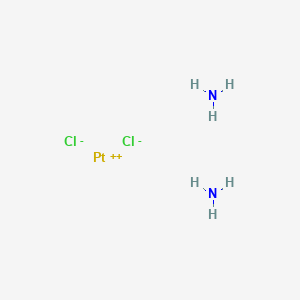
cisplatin
Overview
Description
Scientific Research Applications
Mechanism of Action
Target of Action
Cisplatin primarily targets DNA within the cell . It has a high affinity for the purine bases in DNA, particularly guanine . This makes it highly effective against rapidly proliferating cells, such as cancer cells .
Mode of Action
This compound works by forming covalent bonds with the purine bases in DNA, creating intrastrand and interstrand cross-links . These cross-links distort the DNA structure, inhibiting DNA replication and transcription . This disruption of DNA function triggers apoptosis, or programmed cell death .
Biochemical Pathways
The formation of this compound-DNA adducts initiates several biochemical pathways. These include the activation of signal transduction pathways involving cyclic adenosine monophosphate (cAMP) and protein kinase C (PKC) . These pathways can lead to increased this compound cytotoxicity .
Pharmacokinetics
This compound is administered intravenously and has a bioavailability of 100% . It exhibits biphasic clearance with a rapid initial phase and a prolonged second phase . The primary dose-limiting side effect of this compound is nephrotoxicity, or kidney damage . This is due to the renal accumulation and biotransformation of this compound .
Result of Action
The result of this compound’s action is the inhibition of cancer cell proliferation. By forming covalent bonds with DNA, this compound prevents DNA replication and transcription, leading to cell death . This makes this compound an effective treatment for various types of cancers, including sarcomas, some carcinomas (e.g., small cell lung cancer, and ovarian cancer), lymphomas, and germ cell tumors .
Action Environment
The efficacy and toxicity of this compound can be influenced by the cellular environment. For example, the presence of chloride ions in the cellular environment can affect this compound’s ability to form covalent bonds with DNA . Additionally, factors such as the cell’s detoxification capabilities (e.g., levels of glutathione and metallothionein) and DNA repair mechanisms can influence this compound’s effectiveness and the cell’s resistance to the drug .
Safety and Hazards
Future Directions
The clinical success of Cisplatin is limited due to severe side effects and intrinsic or acquired resistance to the treatment. Much effort has been put into the development of new platinum anticancer complexes . Some new platinum complexes and platinum drug formulations are undergoing clinical trials .
Biochemical Analysis
Biochemical Properties
cis-Diaminodichloroplatinum plays a significant role in biochemical reactions. It crosslinks purine bases within DNA and interferes with DNA synthesis . This impaired cell division is the main effect of cis-Diaminodichloroplatinum, and it shows the highest activity in rapidly proliferating cells .
Cellular Effects
cis-Diaminodichloroplatinum has profound effects on various types of cells and cellular processes. It influences cell function by causing injury to the bone marrow and gastrointestinal tract mucosa due to its high activity in rapidly proliferating cells . The most commonly observed, dose-dependent, and cumulative side effect, noticed in 30–40% of patients, is nephrotoxicity .
Molecular Mechanism
The molecular mechanism of action of cis-Diaminodichloroplatinum is primarily through its ability to form DNA-crosslinks, similar to the group of bifunctional alkylating agents . Cross-resistance is incomplete .
Temporal Effects in Laboratory Settings
The effects of cis-Diaminodichloroplatinum change over time in laboratory settings. The alleviation or prevention of cis-Diaminodichloroplatinum-caused nephrotoxicity is currently accomplished by hydration, magnesium supplementation, or mannitol-induced forced diuresis .
Dosage Effects in Animal Models
The effects of cis-Diaminodichloroplatinum vary with different dosages in animal models. The prominent dose-limiting nephrotoxicity can be effectively circumvented by forced diuresis .
Metabolic Pathways
cis-Diaminodichloroplatinum is involved in various metabolic pathways. It interacts with enzymes and cofactors, causing changes in metabolic flux or metabolite levels .
Transport and Distribution
cis-Diaminodichloroplatinum is transported and distributed within cells and tissues. Its severe side effects, including nephrotoxicity and acute kidney injury, develop due to renal accumulation and biotransformation of cis-Diaminodichloroplatinum .
Subcellular Localization
It is known that cis-Diaminodichloroplatinum causes injury to the bone marrow and gastrointestinal tract mucosa due to its high activity in rapidly proliferating cells .
Preparation Methods
The synthesis of cisplatin involves the reaction of potassium tetrachloroplatinate with ammonia. The process can be summarized as follows :
- Dissolve potassium tetrachloroplatinate in water.
- Add ammonia to the solution, which leads to the formation of cis-Diamminediiodoplatinum.
- The solution is then filtered to remove impurities.
- The filtrate is treated with hydrochloric acid to yield this compound.
Industrial production methods involve similar steps but are scaled up to produce larger quantities. The reaction conditions are carefully controlled to ensure high purity and yield of the final product .
Chemical Reactions Analysis
cisplatin undergoes several types of chemical reactions, including:
Substitution Reactions: The chloride ligands can be substituted by other ligands such as water or ammonia.
Oxidation and Reduction: The platinum center can undergo oxidation and reduction reactions, although these are less common in biological systems.
Common reagents used in these reactions include hydrochloric acid, ammonia, and water. The major products formed depend on the specific reaction conditions but often include various platinum complexes .
Comparison with Similar Compounds
cisplatin is part of a class of platinum-based chemotherapy drugs that includes carboplatin and oxaliplatin . Compared to these compounds, this compound is more potent but also more toxic . Carboplatin has a similar mechanism of action but is less nephrotoxic, making it a preferred option for patients with pre-existing kidney conditions . Oxaliplatin is effective against colorectal cancer and has a different side effect profile, including neurotoxicity .
Similar compounds include:
Carboplatin: Less nephrotoxic, used for ovarian cancer.
Oxaliplatin: Effective against colorectal cancer, causes neurotoxicity.
Nedaplatin: Another platinum-based drug with a different toxicity profile.
This compound remains unique due to its high potency and broad spectrum of activity against various cancers .
Properties
IUPAC Name |
azane;platinum(2+);dichloride | |
---|---|---|
Details | Computed by LexiChem 2.6.6 (PubChem release 2019.06.18) | |
Source | PubChem | |
URL | https://pubchem.ncbi.nlm.nih.gov | |
Description | Data deposited in or computed by PubChem | |
InChI |
InChI=1S/2ClH.2H3N.Pt/h2*1H;2*1H3;/q;;;;+2/p-2 | |
Details | Computed by InChI 1.0.5 (PubChem release 2019.06.18) | |
Source | PubChem | |
URL | https://pubchem.ncbi.nlm.nih.gov | |
Description | Data deposited in or computed by PubChem | |
InChI Key |
LXZZYRPGZAFOLE-UHFFFAOYSA-L | |
Details | Computed by InChI 1.0.5 (PubChem release 2019.06.18) | |
Source | PubChem | |
URL | https://pubchem.ncbi.nlm.nih.gov | |
Description | Data deposited in or computed by PubChem | |
Canonical SMILES |
N.N.[Cl-].[Cl-].[Pt+2] | |
Details | Computed by OEChem 2.1.5 (PubChem release 2019.06.18) | |
Source | PubChem | |
URL | https://pubchem.ncbi.nlm.nih.gov | |
Description | Data deposited in or computed by PubChem | |
Molecular Formula |
Cl2H6N2Pt | |
Details | Computed by PubChem 2.1 (PubChem release 2019.06.18) | |
Source | PubChem | |
URL | https://pubchem.ncbi.nlm.nih.gov | |
Description | Data deposited in or computed by PubChem | |
Molecular Weight |
300.05 g/mol | |
Details | Computed by PubChem 2.1 (PubChem release 2021.08.13) | |
Source | PubChem | |
URL | https://pubchem.ncbi.nlm.nih.gov | |
Description | Data deposited in or computed by PubChem | |
Retrosynthesis Analysis
AI-Powered Synthesis Planning: Our tool employs the Template_relevance Pistachio, Template_relevance Bkms_metabolic, Template_relevance Pistachio_ringbreaker, Template_relevance Reaxys, Template_relevance Reaxys_biocatalysis model, leveraging a vast database of chemical reactions to predict feasible synthetic routes.
One-Step Synthesis Focus: Specifically designed for one-step synthesis, it provides concise and direct routes for your target compounds, streamlining the synthesis process.
Accurate Predictions: Utilizing the extensive PISTACHIO, BKMS_METABOLIC, PISTACHIO_RINGBREAKER, REAXYS, REAXYS_BIOCATALYSIS database, our tool offers high-accuracy predictions, reflecting the latest in chemical research and data.
Strategy Settings
Precursor scoring | Relevance Heuristic |
---|---|
Min. plausibility | 0.01 |
Model | Template_relevance |
Template Set | Pistachio/Bkms_metabolic/Pistachio_ringbreaker/Reaxys/Reaxys_biocatalysis |
Top-N result to add to graph | 6 |
Feasible Synthetic Routes
Disclaimer and Information on In-Vitro Research Products
Please be aware that all articles and product information presented on BenchChem are intended solely for informational purposes. The products available for purchase on BenchChem are specifically designed for in-vitro studies, which are conducted outside of living organisms. In-vitro studies, derived from the Latin term "in glass," involve experiments performed in controlled laboratory settings using cells or tissues. It is important to note that these products are not categorized as medicines or drugs, and they have not received approval from the FDA for the prevention, treatment, or cure of any medical condition, ailment, or disease. We must emphasize that any form of bodily introduction of these products into humans or animals is strictly prohibited by law. It is essential to adhere to these guidelines to ensure compliance with legal and ethical standards in research and experimentation.