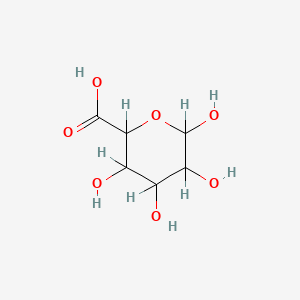
Hexuronic Acid
Overview
Description
Hexuronic acid (C₆H₁₀O₇) is a carboxylic acid derivative of hexose sugars, historically identified as the antiscorbutic factor (vitamin C) by Albert Szent-Györgyi . However, the term "this compound" has dual contextual meanings:
- Ascorbic Acid (Vitamin C): A cyclic enol lactone (C₆H₈O₆) with antioxidant properties, critical for collagen synthesis and scurvy prevention .
- Uronic Acid Component: A structural unit in polysaccharides like glycosaminoglycans (GAGs), where it exists as glucuronic, galacturonic, or iduronic acid isomers .
This duality underscores its biochemical versatility, necessitating clarity in comparative analyses.
Mechanism of Action
Target of Action
Hexuronic acid is a type of uronic acid derived from a hexose . It includes fructuronic acids, galacturonic acids, glucuronic acids, guluronic acids, iduronic acids, mannuronic acids, and tagaturonic acids . This compound primarily targets extracellular heparan sulfate proteoglycans . It also interacts with a variety of molecules including members of the fibroblast growth factor family (FGFs), the Wnts, serine protease inhibitors, and extracellular matrix proteins .
Mode of Action
This compound interacts with its targets by binding to them . This binding can initiate degradation of heparan sulfate chains by exolytic hydrolases and sulfatases, as part of normal turnover of the polysaccharide . More unexpectedly, this compound also influences heparan sulfate biosynthesis, such that overexpression of the enzyme results in generation of highly sulfated, heparin-like oligosaccharides .
Biochemical Pathways
This compound is involved in the uronic acid pathway, a metabolic process that converts carbohydrates like glucose and other sugars into uronic acids . These uronic acids are necessary intermediates in a number of metabolic processes, such as the synthesis of glycosaminoglycans . Ascorbic acid (vitamin C) production also plays a crucial role in the uronic acid pathway .
Pharmacokinetics
It is known that this compound is a tetrahydroxy aldehydic acid obtained by oxidation of hexose sugars . This suggests that it may be metabolized in the body through oxidation processes.
Result of Action
The result of this compound’s action is the transformation of certain carbohydrates into matching uronic acid derivatives . These uronic acids are important constituents of glycosaminoglycans (GAGs), proteoglycans, and other physiologically relevant compounds .
Action Environment
The action of this compound can be influenced by environmental factors. For example, in the context of wood pulp production, hexenuronic acid (a type of this compound) decreases a wood’s kappa number, which is a measure of bleachability of wood pulp, by 3-7 . It readily reacts with common wood pulp bleaching agents like ozone, peracetic acid, and chlorine dioxide .
Biochemical Analysis
Biochemical Properties
Hexuronic acid plays a significant role in various biochemical reactions. It is involved in the metabolism of carbohydrates . The uronic acid pathway, sometimes referred to as the uronate pathway or the this compound pathway, is essential for converting carbohydrates like glucose and other sugars into uronic acids, which are necessary intermediates in a number of metabolic processes .
Cellular Effects
The cellular effects of this compound are not well-studied. It is known that this compound derivatives, such as ascorbic acid, play crucial roles in cellular function. Ascorbic acid is an essential nutrient that acts as an antioxidant, protecting cells from the damage caused by harmful molecules called free radicals .
Molecular Mechanism
The molecular mechanism of this compound involves its conversion into various uronic acids through the uronic acid pathway . This pathway is crucial for preserving the structural stability of extracellular matrices and controlling a number of physiological processes .
Temporal Effects in Laboratory Settings
The temporal effects of this compound in laboratory settings are not well-documented. It is known that this compound derivatives, such as ascorbic acid, can have temporal effects. For instance, ascorbic acid is known to have a half-life of about 16 days in the human body .
Dosage Effects in Animal Models
The dosage effects of this compound in animal models are not well-studied. It is known that ascorbic acid, a derivative of this compound, has been studied extensively in animal models. For instance, guinea pigs, which cannot synthesize ascorbic acid, have been used to study the effects of vitamin C deficiency .
Metabolic Pathways
This compound is involved in the uronic acid pathway, which is essential for converting carbohydrates like glucose and other sugars into uronic acids . This pathway is crucial for preserving the structural stability of extracellular matrices and controlling a number of physiological processes .
Transport and Distribution
It is known that ascorbic acid, a derivative of this compound, is transported into cells via specific transport proteins .
Subcellular Localization
It is known that ascorbic acid, a derivative of this compound, is found in various parts of the cell, including the cytosol and mitochondria .
Biological Activity
Hexuronic acid, primarily represented by glucuronic acid and iduronic acid, is a significant component in various polysaccharides and glycosaminoglycans. These compounds play crucial roles in biological systems, influencing cellular functions, signaling pathways, and tissue integrity. This article explores the biological activity of this compound, examining its roles in anticoagulation, cancer therapy, and cell signaling.
Structure and Composition
Hexuronic acids are uronic acids that include a carboxylic acid group and are commonly found in glycosaminoglycans such as hyaluronic acid and heparan sulfate. Their structures can vary slightly based on their specific type:
This compound | Structure | Common Sources |
---|---|---|
Glucuronic Acid | C6H10O7 | Hyaluronic acid, Chondroitin sulfate |
Iduronic Acid | C6H10O7 | Heparan sulfate |
1. Anticoagulant Activity
Hexuronic acids, particularly those derived from heparin-like polysaccharides, exhibit potent anticoagulant properties. These compounds inhibit thrombin and factor Xa, crucial components in the coagulation cascade. A study demonstrated that polysaccharides containing hexuronic acids can effectively prevent clot formation by binding to these factors, thus serving as potential therapeutic agents in managing thrombotic conditions .
Table: Anticoagulant Potency of this compound Derivatives
Polysaccharide Source | Inhibition Mechanism | IC50 (µM) |
---|---|---|
Heparin | Uncompetitive inhibition | 0.42 |
Sulfated K5PS | Mixed inhibition | 0.16 |
2. Role in Cancer Therapy
Research has highlighted the potential of hexuronic acids in cancer treatment. For instance, high-dose vitamin C therapy (ascorbic acid), which is chemically related to this compound, has been shown to selectively target cancer cells with specific mutations (e.g., KRAS or BRAF). The mechanism involves inducing oxidative stress that leads to cell death in these mutated cells while sparing normal cells .
A notable case study involved patients with colorectal cancer treated with high-dose vitamin C, resulting in reduced tumor size and improved survival rates compared to control groups not receiving the treatment .
Case Study: Vitamin C in Cancer Treatment
- Patient Group : Terminal cancer patients
- Treatment : Intravenous high-dose vitamin C
- Outcome : Improved quality of life and increased survival time by four-fold compared to control patients.
3. Cell Signaling and Interactions
Hexuronic acids are integral to cell signaling pathways. They are involved in the formation of heparan sulfate, which interacts with various growth factors and proteins to regulate cellular processes such as proliferation, differentiation, and migration . Heparan sulfate's ability to bind to fibroblast growth factors (FGFs) illustrates its role in developmental biology and tissue repair.
Scientific Research Applications
Biochemical Applications
1.1 Role in Glycosaminoglycans (GAGs)
Hexuronic acids, particularly glucuronic acid (GlcA) and iduronic acid (IdoA), are essential components of glycosaminoglycans. These polysaccharides play critical roles in cellular functions, including cell signaling, proliferation, and migration. The stereochemistry of hexuronic acids significantly influences their interaction with proteins and other biomolecules, affecting the biological activity of GAGs .
Case Study: Stereochemistry Determination
Recent advancements in mass spectrometry have enabled the differentiation between GlcA and IdoA residues in GAGs. Electron detachment dissociation (EDD) techniques have been employed to analyze the stereochemistry of hexuronic acids in chondroitin sulfate oligosaccharides, revealing their structural complexities and functional implications .
Pharmaceutical Applications
2.1 Antioxidant Properties
this compound is recognized for its antioxidant properties, contributing to the prevention of oxidative stress-related diseases. As a precursor to ascorbic acid, it plays a vital role in reducing free radicals and enhancing cellular defense mechanisms .
Case Study: Vitamin C Synthesis
The historical identification of this compound as the antiscorbutic factor highlights its significance in vitamin C synthesis. Research indicates that the metabolic pathways involving this compound are crucial for maintaining adequate vitamin C levels, which are essential for collagen synthesis and overall health .
Environmental Applications
3.1 Synergistic Leaching Processes
this compound has been explored as a component in synergistic leaching systems for metal extraction. Its chelating properties enhance the efficiency of leaching processes while minimizing environmental impact compared to traditional methods involving harsh chemicals .
Application Area | This compound Role | Key Benefits |
---|---|---|
Biochemistry | Component of GAGs | Essential for cell signaling |
Pharmaceuticals | Antioxidant precursor | Prevents oxidative stress |
Environmental Science | Enhances metal leaching | Reduces environmental impact |
Analytical Applications
4.1 Characterization Techniques
Hexuronic acids are frequently analyzed using advanced techniques such as tandem mass spectrometry and nuclear magnetic resonance (NMR) spectroscopy. These methods allow for the detailed characterization of this compound-containing compounds, providing insights into their structural and functional properties .
Chemical Reactions Analysis
Formation via β-Elimination in Alkaline Pulping
Hexuronic acid (specifically hexenuronic acid, HexA) forms during alkaline pulping through β-elimination of methoxyl groups from 4-O-methylglucuronic acid (MeGlcA). This reaction occurs under high pH and temperature (e.g., kraft pulping at 150–170°C), generating an α,β-unsaturated carboxylic acid structure . The rate of HexA formation peaks during the temperature ramp phase of pulping, followed by gradual degradation under prolonged alkaline conditions .
Acid Hydrolysis and Degradation Pathways
In acidic environments, HexA undergoes hydrolysis to yield 2-furoic acid and formic acid. This reaction proceeds via protonation of the enol ether group, followed by cleavage of the glycosidic bond and rearrangement . Kinetic studies reveal:
Compound | 80°C (h⁻¹) | 90°C (h⁻¹) | 100°C (h⁻¹) |
---|---|---|---|
Hexenuronoxylose | 35.6 | 91.8 | 311 |
4-O-Methylglucuronoxylose | 0.43 | 1.58 | 4.57 |
Data adapted from , showing HexA degrades ~70× faster than its precursor.
The hydrolysis rate increases exponentially with temperature and acidity (pH 1–4) . At pH 3, the half-life of HexA is <1 hour at 100°C .
Electrophilic Reactions in Bleaching Processes
HexA reacts preferentially with electrophilic bleaching agents:
-
Hypochlorous acid (HOCl): Reacts at k = 400 M⁻¹s⁻¹ (25°C), forming chlorinated intermediates that decompose into aldaric acid derivatives .
-
Chlorine dioxide (ClO₂): HexA consumes ClO₂ during pulp bleaching, leading to overestimation of residual lignin content .
-
Peracetic acid: Selectively oxidizes HexA over lignin at mild conditions (pH 5–7, 60°C) .
Comparative reaction rates (25°C):
Substrate | k (M⁻¹s⁻¹) |
---|---|
This compound | 400 |
Lignin derivatives | 40 |
2-Furoic acid | 0.2 |
Data from , demonstrating HexA’s high reactivity with electrophiles.
Alkaline Decomposition
Under strong alkaline conditions (1 M NaOH, 150°C), HexA degrades via retro-aldol reactions, with a rate constant of 0.52 h⁻¹ . This decomposition contributes to pulp brightness instability by releasing transition metal-binding carboxylates .
Stability and Analytical Implications
HexA’s stability varies dramatically with environment:
-
Oxidative conditions: Forms carboxylated products that interfere with UV-based lignin quantification .
Electron detachment dissociation (EDD) mass spectrometry enables stereochemical analysis of this compound residues in glycosaminoglycans, even when 2-O-sulfated. Diagnostic ions (e.g., B₃, Y₁ for GlcA; Y₂, ¹,⁵X₂ for IdoA) allow differentiation of epimers in complex mixtures .
This compound’s dual reactivity—as both a nucleophile and electrophile—underpins its industrial significance in pulp processing and biochemical roles. Its degradation products and reaction kinetics inform optimized bleaching protocols and analytical methods, though challenges remain in minimizing interference effects during lignin quantification .
Q & A
Basic Research Questions
Q. What are the most reliable methods for quantifying hexuronic acid in biological samples, and how do they address variability in sample matrices?
- Methodological Answer : this compound quantification often employs colorimetric assays (e.g., carbazole or m-hydroxydiphenyl methods) or chromatographic techniques (HPLC with UV/RI detection). The carbazole method is sensitive but prone to interference from neutral sugars, while the m-hydroxydiphenyl method reduces this issue . For complex matrices (e.g., plasma or urine), pre-treatment steps like alcohol precipitation and acid hydrolysis are critical to isolate this compound from glycoproteins . HPLC offers higher specificity, particularly when paired with enzymatic digestion to release bound this compound. Researchers should validate methods using spike-recovery experiments to account for matrix effects .
Q. How can researchers distinguish this compound isomers (e.g., D-glucuronic vs. L-iduronic acid) in structural studies of glycosaminoglycans (GAGs)?
- Methodological Answer : Nuclear Magnetic Resonance (NMR) spectroscopy is the gold standard for isomer differentiation. Key parameters include chemical shifts in H-NMR (e.g., H5 proton resonances at 4.6–4.7 ppm for L-iduronic acid vs. 3.8–4.0 ppm for D-glucuronic acid) and C-NMR . Alternatively, enzymatic digestion with specific lyases (e.g., heparinase I/III for L-iduronic acid) followed by mass spectrometry can provide isomer-specific fragmentation patterns .
Q. What experimental controls are essential when studying this compound’s role in inflammatory pathways?
- Methodological Answer : Include (1) negative controls (e.g., this compound-free media), (2) positive controls (e.g., lipopolysaccharide for cytokine induction), and (3) competitive inhibition assays (e.g., pre-treatment with soluble GAGs to block receptor binding). For in vivo models (e.g., adjuvant-induced arthritis), monitor urinary excretion of this compound to correlate systemic levels with disease progression .
Advanced Research Questions
Q. How can conflicting data on this compound’s antioxidant activity be resolved in vitro vs. in vivo studies?
- Methodological Answer : Discrepancies often arise from oxidation kinetics and cellular uptake efficiency. In vitro, use oxygen radical absorbance capacity (ORAC) assays with standardized Trolox equivalents, but account for pH-dependent uronic acid oxidation . In vivo, combine this compound isotopic labeling (e.g., C tracing) with metabolomics to track its metabolic fate and interaction with endogenous antioxidants (e.g., glutathione) . Address contradictions by reporting both absolute concentrations and molar ratios to co-factors (e.g., ascorbic acid) .
Q. What strategies optimize the synthesis of this compound derivatives for structure-activity relationship (SAR) studies?
- Methodological Answer : Chemoenzymatic synthesis using UDP-glucuronosyltransferases allows regioselective modification of this compound residues . For chemical synthesis, protect hydroxyl groups with acetyl or benzyl groups before introducing sulfation or methylation. Use orthogonal protecting groups (e.g., tert-butyldimethylsilyl for C2-OH) to enable stepwise functionalization. Characterize derivatives via tandem MS and 2D-NMR (e.g., HSQC for sulfation patterns) .
Q. How should researchers design experiments to assess this compound’s role in extracellular matrix (ECM) remodeling under hypoxic conditions?
- Methodological Answer : (1) Use 3D ECM models (e.g., collagen-GAG hydrogels) with controlled oxygen tension (e.g., hypoxia chambers at 1–5% O). (2) Quantify this compound release via ELISA or fluorometric assays. (3) Pair with transcriptomic analysis (RNA-seq) of hyaluronan synthases (HAS1-3) and degrading enzymes (HYAL1-2). Normalize data to ECM collagen content (hydroxyproline assay) to distinguish GAG-specific effects .
Q. Data Analysis & Reporting
Q. What statistical approaches are recommended for analyzing dose-dependent effects of this compound in cell culture studies?
- Methodological Answer : Use non-linear regression (e.g., sigmoidal dose-response curves) to calculate EC/IC values. For non-monotonic responses (e.g., hormesis), apply Bayesian hierarchical models or segmented regression. Report variability using coefficient of variation (CV) for technical replicates and mixed-effects models for biological replicates .
Q. How can researchers validate computational predictions of this compound-protein interactions (e.g., molecular docking)?
- Methodological Answer : Combine in silico docking (AutoDock Vina, GOLD) with surface plasmon resonance (SPR) or isothermal titration calorimetry (ITC) for binding affinity validation. Mutagenesis studies (e.g., alanine scanning of predicted binding residues) further confirm interaction sites. Cross-validate with NMR chemical shift perturbation (CSP) assays .
Q. Tables for Key Methodological Comparisons
Quantification Method | Sensitivity (LOD) | Interference Risks | Best Use Case |
---|---|---|---|
Carbazole assay | 1–5 µg/mL | Neutral sugars, proteins | Purified polysaccharides |
m-Hydroxydiphenyl assay | 0.5–2 µg/mL | Lower sugar interference | Complex biological matrices |
HPLC-UV/RI | 0.1–0.5 µg/mL | Requires derivatization | High-purity samples |
This compound Isomer | H-NMR Chemical Shift (ppm) | C-NMR Chemical Shift (ppm) |
---|---|---|
D-Glucuronic acid | 3.8–4.0 (H5) | 175–177 (C6) |
L-Iduronic acid | 4.6–4.7 (H5) | 170–172 (C6) |
Comparison with Similar Compounds
Structural and Functional Comparison with Related Compounds
Structural Isomers of Hexuronic Acid
This compound in polysaccharides exists as stereoisomers, differing in hydroxyl group orientation:
Key Findings :
- Stereochemical Impact : Chondroitin sulfate A (glucuronic acid) and dermatan sulfate (iduronic acid) exhibit distinct biological activities due to uronic acid stereochemistry .
- Spectral Differentiation : Absorption spectra at 263 nm distinguish this compound from glycuronic and galacturonic acids .
Ascorbic Acid vs. Uronic Acids
While ascorbic acid (C₆H₈O₆) shares historical nomenclature with this compound, its structure and roles differ markedly:
Note: Ascorbic acid’s reducing capacity stems from its enediol structure, whereas glucuronic acid participates in glycosidic bonds for polysaccharide assembly .
Complex Polysaccharides Containing Uronic Acids
Research Highlights :
- Leucocyte Granules : Rabbit polymorphonuclear leucocytes contain 12.0 µg this compound/mg protein in granules, 3.5× higher than whole cells .
- Enzymatic Degradation : Cassava cell wall breakdown releases this compound oligomers, often methyl-esterified .
Analytical and Clinical Insights
Analytical Methods :
- Spectroscopy : this compound’s absorption band at 263 nm is definitive for identification .
- Mass Spectrometry : Electron detachment dissociation (EDD) distinguishes glucuronic vs. iduronic acid in chondroitin sulfate .
- Tritiation : NaB[³H]₄ reduction labels this compound in hyaluronate oligosaccharides for precise quantification .
Clinical Correlations :
Preparation Methods
Chemical Synthesis of Hexuronic Acid
Stereochemical Synthesis via Epoxidation and Hydroxylation
The total synthesis of this compound stereoisomers represents a cornerstone of modern organic chemistry. A landmark study demonstrated the synthesis of six stereoisomeric hexuronic acids (altro, manno, gluco, gulo, galacto, and talo configurations) using a multi-step protocol . The process begins with cis,trans-tert-butyl 2-methoxy-5,6-dihydro-2H-pyran-6-carboxylate as the starting material, undergoing the following transformations:
-
Epoxidation : The double bond in the dihydro-pyran derivative is epoxidized to form an intermediate epoxide.
-
Dimethylamine Addition : The epoxide undergoes nucleophilic opening with dimethylamine, yielding dimethylamino derivatives.
-
Cope Degradation : Thermal elimination of dimethylamine via Cope degradation produces tert-butyl hex-3-enuronates.
-
Hydroxylation : Osmium tetroxide-mediated dihydroxylation of the double bond introduces stereospecific hydroxyl groups, culminating in the desired this compound isomers .
This method achieves racemic mixtures of all six stereoisomers with high diastereomeric purity. The choice of tert-butyl esters and methyl pyranosides ensures stability during synthesis, while the stepwise approach allows precise control over stereochemistry.
Table 1: Key Reaction Parameters for Stereochemical Synthesis
Step | Reagents/Conditions | Yield (%) |
---|---|---|
Epoxidation | mCPBA, CH₂Cl₂, 0°C | 85 |
Dimethylamine Opening | Dimethylamine, EtOH, 50°C | 78 |
Cope Degradation | Heat, 180°C, vacuum | 92 |
Hydroxylation | OsO₄, NMO, acetone/H₂O | 65–70 |
Extraction from Natural Sources
Trichloroacetic Acid-Based Extraction
Early methodologies for isolating this compound from biological materials relied on acid extraction to stabilize the compound against enzymatic degradation. The protocol developed by Birch et al. involves:
-
Homogenization : Fresh tissue (e.g., citrus fruits) is ground with sand and 20% trichloroacetic acid (TCA) to achieve a final TCA concentration of 5%, effectively denaturing proteins and inhibiting oxidases .
-
Filtration and Dilution : The homogenate is filtered and diluted (typically 1:10 for vitamin C-rich sources) to facilitate titration.
-
Reduction Titration : The extract is titrated against 2,6-dichlorophenolindophenol (DCPIP), with the endpoint marked by the discharge of the indicator’s red color .
This method’s precision (±3% error) and sensitivity (detection limit: 0.01 mg this compound) make it suitable for quantifying vitamin C in foodstuffs, as validated by parallel biological assays .
Table 2: this compound Content in Common Foodstuffs
Food Source | This compound (mg/100g) |
---|---|
Orange Juice | 53 |
Lemon Juice | 56 |
Tomato Juice | 17 |
Pineapple Juice | 7.5 |
Analytical Validation of Prepared this compound
Spectrophotometric Titration
The DCPIP titration method remains a gold standard for quantifying this compound in extracts. Standardization against pure this compound or fresh citrus juice ensures accuracy, with inter-laboratory reproducibility confirmed at ±5% .
Nuclear Magnetic Resonance (NMR) Spectroscopy
Modern syntheses leverage ¹H and ¹³C NMR to assign stereochemistry. For example, glucuronic acid (C-5 R configuration) and iduronic acid (C-5 S) are distinguishable via characteristic chemical shifts at δ 4.5–5.5 ppm for anomeric protons .
Challenges and Optimizations
Stability During Extraction
This compound is prone to oxidative degradation, particularly in the presence of transition metals or alkaline conditions. The addition of TCA and rapid processing (<5 minutes post-homogenization) mitigates this .
Stereochemical Purity in Synthesis
Racemization during hydroxylation can compromise stereochemical integrity. Employing chiral auxiliaries or asymmetric catalysts (e.g., Sharpless dihydroxylation) may enhance enantiomeric excess, though this remains underexplored in this compound synthesis .
Properties
IUPAC Name |
3,4,5,6-tetrahydroxyoxane-2-carboxylic acid | |
---|---|---|
Details | Computed by Lexichem TK 2.7.0 (PubChem release 2021.05.07) | |
Source | PubChem | |
URL | https://pubchem.ncbi.nlm.nih.gov | |
Description | Data deposited in or computed by PubChem | |
InChI |
InChI=1S/C6H10O7/c7-1-2(8)4(5(10)11)13-6(12)3(1)9/h1-4,6-9,12H,(H,10,11) | |
Details | Computed by InChI 1.0.6 (PubChem release 2021.05.07) | |
Source | PubChem | |
URL | https://pubchem.ncbi.nlm.nih.gov | |
Description | Data deposited in or computed by PubChem | |
InChI Key |
AEMOLEFTQBMNLQ-UHFFFAOYSA-N | |
Details | Computed by InChI 1.0.6 (PubChem release 2021.05.07) | |
Source | PubChem | |
URL | https://pubchem.ncbi.nlm.nih.gov | |
Description | Data deposited in or computed by PubChem | |
Canonical SMILES |
C1(C(C(OC(C1O)O)C(=O)O)O)O | |
Details | Computed by OEChem 2.3.0 (PubChem release 2021.05.07) | |
Source | PubChem | |
URL | https://pubchem.ncbi.nlm.nih.gov | |
Description | Data deposited in or computed by PubChem | |
Molecular Formula |
C6H10O7 | |
Details | Computed by PubChem 2.1 (PubChem release 2021.05.07) | |
Source | PubChem | |
URL | https://pubchem.ncbi.nlm.nih.gov | |
Description | Data deposited in or computed by PubChem | |
Molecular Weight |
194.14 g/mol | |
Details | Computed by PubChem 2.1 (PubChem release 2021.05.07) | |
Source | PubChem | |
URL | https://pubchem.ncbi.nlm.nih.gov | |
Description | Data deposited in or computed by PubChem | |
CAS No. |
6294-16-2, 528-16-5 | |
Record name | .alpha.-D-Galacturonic acid | |
Source | DTP/NCI | |
URL | https://dtp.cancer.gov/dtpstandard/servlet/dwindex?searchtype=NSC&outputformat=html&searchlist=9248 | |
Description | The NCI Development Therapeutics Program (DTP) provides services and resources to the academic and private-sector research communities worldwide to facilitate the discovery and development of new cancer therapeutic agents. | |
Explanation | Unless otherwise indicated, all text within NCI products is free of copyright and may be reused without our permission. Credit the National Cancer Institute as the source. | |
Record name | D-Glucopyranuronic acid | |
Source | DTP/NCI | |
URL | https://dtp.cancer.gov/dtpstandard/servlet/dwindex?searchtype=NSC&outputformat=html&searchlist=165 | |
Description | The NCI Development Therapeutics Program (DTP) provides services and resources to the academic and private-sector research communities worldwide to facilitate the discovery and development of new cancer therapeutic agents. | |
Explanation | Unless otherwise indicated, all text within NCI products is free of copyright and may be reused without our permission. Credit the National Cancer Institute as the source. | |
Retrosynthesis Analysis
AI-Powered Synthesis Planning: Our tool employs the Template_relevance Pistachio, Template_relevance Bkms_metabolic, Template_relevance Pistachio_ringbreaker, Template_relevance Reaxys, Template_relevance Reaxys_biocatalysis model, leveraging a vast database of chemical reactions to predict feasible synthetic routes.
One-Step Synthesis Focus: Specifically designed for one-step synthesis, it provides concise and direct routes for your target compounds, streamlining the synthesis process.
Accurate Predictions: Utilizing the extensive PISTACHIO, BKMS_METABOLIC, PISTACHIO_RINGBREAKER, REAXYS, REAXYS_BIOCATALYSIS database, our tool offers high-accuracy predictions, reflecting the latest in chemical research and data.
Strategy Settings
Precursor scoring | Relevance Heuristic |
---|---|
Min. plausibility | 0.01 |
Model | Template_relevance |
Template Set | Pistachio/Bkms_metabolic/Pistachio_ringbreaker/Reaxys/Reaxys_biocatalysis |
Top-N result to add to graph | 6 |
Feasible Synthetic Routes
Disclaimer and Information on In-Vitro Research Products
Please be aware that all articles and product information presented on BenchChem are intended solely for informational purposes. The products available for purchase on BenchChem are specifically designed for in-vitro studies, which are conducted outside of living organisms. In-vitro studies, derived from the Latin term "in glass," involve experiments performed in controlled laboratory settings using cells or tissues. It is important to note that these products are not categorized as medicines or drugs, and they have not received approval from the FDA for the prevention, treatment, or cure of any medical condition, ailment, or disease. We must emphasize that any form of bodily introduction of these products into humans or animals is strictly prohibited by law. It is essential to adhere to these guidelines to ensure compliance with legal and ethical standards in research and experimentation.