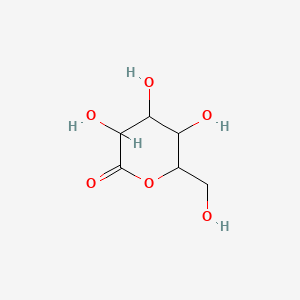
D-Gluconic acid, delta-lactone
Overview
Description
D-Gluconic acid, delta-lactone: . It is a white, odorless crystalline powder that is soluble in water. This compound is an oxidized derivative of glucose and is commonly used in the food industry as a sequestrant, acidifier, and leavening agent .
Preparation Methods
Synthetic Routes and Reaction Conditions: : D-Gluconic acid, delta-lactone is typically produced by the aerobic oxidation of glucose in the presence of the enzyme glucose oxidase . The reaction can be represented as follows: [ \text{C}6\text{H}{12}\text{O}_6 + \text{O}_2 \rightarrow \text{C}6\text{H}{10}\text{O}_6 + \text{H}_2\text{O}_2 ]
Industrial Production Methods: : Industrial production of this compound involves the direct crystallization from the aqueous solution of gluconic acid . The process is efficient and yields high-purity glucono delta-lactone.
Chemical Reactions Analysis
Types of Reactions
-
Hydrolysis: : D-Gluconic acid, delta-lactone spontaneously hydrolyzes to form gluconic acid in the presence of water . [ \text{C}6\text{H}{10}\text{O}_6 + \text{H}_2\text{O} \rightarrow \text{C}6\text{H}{12}\text{O}_7 ]
-
Oxidation: : It can undergo further oxidation to form other derivatives, such as 6-phospho-D-gluconate .
Common Reagents and Conditions
Water: For hydrolysis reactions.
Enzymes: Such as glucose oxidase for oxidation reactions.
Major Products
Gluconic acid: Formed through hydrolysis.
6-Phospho-D-gluconate: Formed through enzymatic oxidation.
Scientific Research Applications
Chemistry
Biology
Medicine
Industry
Mechanism of Action
D-Gluconic acid, delta-lactone exerts its effects primarily through hydrolysis to gluconic acid, which lowers the pH of the surrounding environment . This acidification process is crucial in various applications, such as food preservation and gel formation. The compound is metabolized to 6-phospho-D-gluconate, which plays a role in metabolic pathways .
Comparison with Similar Compounds
Similar Compounds
- Gluconolactone
- 1,2,3,4,5-Pentahydroxycaproic acid delta-lactone
- D- (+)-Dextronic acid delta-lactone
Uniqueness: : D-Gluconic acid, delta-lactone is unique due to its ability to hydrolyze spontaneously to gluconic acid, making it a versatile compound in various applications. Its pH-neutral nature, which changes upon hydrolysis, adds to its distinctiveness .
Properties
IUPAC Name |
3,4,5-trihydroxy-6-(hydroxymethyl)oxan-2-one | |
---|---|---|
Details | Computed by Lexichem TK 2.7.0 (PubChem release 2021.05.07) | |
Source | PubChem | |
URL | https://pubchem.ncbi.nlm.nih.gov | |
Description | Data deposited in or computed by PubChem | |
InChI |
InChI=1S/C6H10O6/c7-1-2-3(8)4(9)5(10)6(11)12-2/h2-5,7-10H,1H2 | |
Details | Computed by InChI 1.0.6 (PubChem release 2021.05.07) | |
Source | PubChem | |
URL | https://pubchem.ncbi.nlm.nih.gov | |
Description | Data deposited in or computed by PubChem | |
InChI Key |
PHOQVHQSTUBQQK-UHFFFAOYSA-N | |
Details | Computed by InChI 1.0.6 (PubChem release 2021.05.07) | |
Source | PubChem | |
URL | https://pubchem.ncbi.nlm.nih.gov | |
Description | Data deposited in or computed by PubChem | |
Canonical SMILES |
C(C1C(C(C(C(=O)O1)O)O)O)O | |
Details | Computed by OEChem 2.3.0 (PubChem release 2021.05.07) | |
Source | PubChem | |
URL | https://pubchem.ncbi.nlm.nih.gov | |
Description | Data deposited in or computed by PubChem | |
Molecular Formula |
C6H10O6 | |
Details | Computed by PubChem 2.1 (PubChem release 2021.05.07) | |
Source | PubChem | |
URL | https://pubchem.ncbi.nlm.nih.gov | |
Description | Data deposited in or computed by PubChem | |
DSSTOX Substance ID |
DTXSID20859158 | |
Record name | 3,4,5-Trihydroxy-6-(hydroxymethyl)oxan-2-one (non-preferred name) | |
Source | EPA DSSTox | |
URL | https://comptox.epa.gov/dashboard/DTXSID20859158 | |
Description | DSSTox provides a high quality public chemistry resource for supporting improved predictive toxicology. | |
Molecular Weight |
178.14 g/mol | |
Details | Computed by PubChem 2.1 (PubChem release 2021.05.07) | |
Source | PubChem | |
URL | https://pubchem.ncbi.nlm.nih.gov | |
Description | Data deposited in or computed by PubChem | |
Physical Description |
Dry Powder | |
Record name | D-Gluconic acid, .delta.-lactone | |
Source | EPA Chemicals under the TSCA | |
URL | https://www.epa.gov/chemicals-under-tsca | |
Description | EPA Chemicals under the Toxic Substances Control Act (TSCA) collection contains information on chemicals and their regulations under TSCA, including non-confidential content from the TSCA Chemical Substance Inventory and Chemical Data Reporting. | |
CAS No. |
54910-68-8, 7506-65-2, 90-80-2 | |
Record name | D-Gluconic acid, delta-lactone | |
Source | ChemIDplus | |
URL | https://pubchem.ncbi.nlm.nih.gov/substance/?source=chemidplus&sourceid=0054910688 | |
Description | ChemIDplus is a free, web search system that provides access to the structure and nomenclature authority files used for the identification of chemical substances cited in National Library of Medicine (NLM) databases, including the TOXNET system. | |
Record name | Galactonic acid, 8CI, | |
Source | DTP/NCI | |
URL | https://dtp.cancer.gov/dtpstandard/servlet/dwindex?searchtype=NSC&outputformat=html&searchlist=407014 | |
Description | The NCI Development Therapeutics Program (DTP) provides services and resources to the academic and private-sector research communities worldwide to facilitate the discovery and development of new cancer therapeutic agents. | |
Explanation | Unless otherwise indicated, all text within NCI products is free of copyright and may be reused without our permission. Credit the National Cancer Institute as the source. | |
Record name | NSC34393 | |
Source | DTP/NCI | |
URL | https://dtp.cancer.gov/dtpstandard/servlet/dwindex?searchtype=NSC&outputformat=html&searchlist=34393 | |
Description | The NCI Development Therapeutics Program (DTP) provides services and resources to the academic and private-sector research communities worldwide to facilitate the discovery and development of new cancer therapeutic agents. | |
Explanation | Unless otherwise indicated, all text within NCI products is free of copyright and may be reused without our permission. Credit the National Cancer Institute as the source. | |
Record name | D-Gluconic acid, .delta.-lactone | |
Source | EPA Chemicals under the TSCA | |
URL | https://www.epa.gov/chemicals-under-tsca | |
Description | EPA Chemicals under the Toxic Substances Control Act (TSCA) collection contains information on chemicals and their regulations under TSCA, including non-confidential content from the TSCA Chemical Substance Inventory and Chemical Data Reporting. | |
Record name | 3,4,5-Trihydroxy-6-(hydroxymethyl)oxan-2-one (non-preferred name) | |
Source | EPA DSSTox | |
URL | https://comptox.epa.gov/dashboard/DTXSID20859158 | |
Description | DSSTox provides a high quality public chemistry resource for supporting improved predictive toxicology. | |
Retrosynthesis Analysis
AI-Powered Synthesis Planning: Our tool employs the Template_relevance Pistachio, Template_relevance Bkms_metabolic, Template_relevance Pistachio_ringbreaker, Template_relevance Reaxys, Template_relevance Reaxys_biocatalysis model, leveraging a vast database of chemical reactions to predict feasible synthetic routes.
One-Step Synthesis Focus: Specifically designed for one-step synthesis, it provides concise and direct routes for your target compounds, streamlining the synthesis process.
Accurate Predictions: Utilizing the extensive PISTACHIO, BKMS_METABOLIC, PISTACHIO_RINGBREAKER, REAXYS, REAXYS_BIOCATALYSIS database, our tool offers high-accuracy predictions, reflecting the latest in chemical research and data.
Strategy Settings
Precursor scoring | Relevance Heuristic |
---|---|
Min. plausibility | 0.01 |
Model | Template_relevance |
Template Set | Pistachio/Bkms_metabolic/Pistachio_ringbreaker/Reaxys/Reaxys_biocatalysis |
Top-N result to add to graph | 6 |
Feasible Synthetic Routes
Disclaimer and Information on In-Vitro Research Products
Please be aware that all articles and product information presented on BenchChem are intended solely for informational purposes. The products available for purchase on BenchChem are specifically designed for in-vitro studies, which are conducted outside of living organisms. In-vitro studies, derived from the Latin term "in glass," involve experiments performed in controlled laboratory settings using cells or tissues. It is important to note that these products are not categorized as medicines or drugs, and they have not received approval from the FDA for the prevention, treatment, or cure of any medical condition, ailment, or disease. We must emphasize that any form of bodily introduction of these products into humans or animals is strictly prohibited by law. It is essential to adhere to these guidelines to ensure compliance with legal and ethical standards in research and experimentation.