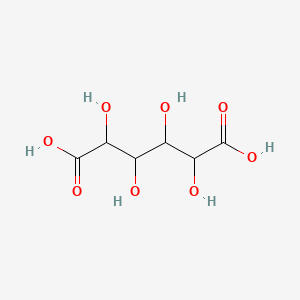
L-idaric acid
Overview
Description
Obetrol was a pharmaceutical drug that combined several amphetamine salts. It was originally marketed by Obetrol Pharmaceuticals in the 1950s and 1960s for the treatment of exogenous obesity. The drug contained a mixture of methamphetamine saccharate, methamphetamine hydrochloride, racemic amphetamine sulfate, and dextroamphetamine sulfate . Obetrol was later reformulated and rebranded as Adderall .
Preparation Methods
Synthetic Routes and Reaction Conditions
The preparation of Obetrol involved the synthesis of its constituent amphetamine salts. The synthetic routes for these compounds typically involve the following steps:
Methamphetamine Saccharate: This is synthesized by the reduction of ephedrine or pseudoephedrine using a reducing agent such as red phosphorus and iodine or lithium aluminum hydride.
Methamphetamine Hydrochloride: This is prepared by the reaction of methamphetamine base with hydrochloric acid.
Racemic Amphetamine Sulfate: This is synthesized by the condensation of phenylacetone with ammonia, followed by reduction and subsequent reaction with sulfuric acid.
Dextroamphetamine Sulfate: This is prepared by the resolution of racemic amphetamine using tartaric acid to separate the dextro and levo isomers, followed by reaction with sulfuric acid.
Industrial Production Methods
Industrial production of Obetrol involved large-scale synthesis of the individual amphetamine salts, followed by their combination in specific proportions. The process required strict adherence to safety and regulatory guidelines due to the controlled nature of the substances involved .
Chemical Reactions Analysis
Types of Reactions
Obetrol and its constituent compounds undergo various chemical reactions, including:
Oxidation: Amphetamines can be oxidized to form corresponding oximes and nitro compounds.
Reduction: Reduction of ephedrine or pseudoephedrine to methamphetamine.
Substitution: Halogenation and nitration reactions on the aromatic ring of amphetamines.
Common Reagents and Conditions
Oxidation: Reagents such as potassium permanganate or chromium trioxide.
Reduction: Reducing agents like lithium aluminum hydride or red phosphorus and iodine.
Substitution: Halogenating agents like chlorine or bromine, and nitrating agents like nitric acid.
Major Products
Oxidation: Formation of oximes and nitro compounds.
Reduction: Formation of methamphetamine from ephedrine or pseudoephedrine.
Substitution: Formation of halogenated or nitrated amphetamines.
Scientific Research Applications
Obetrol has been studied for its effects on the central nervous system and its potential applications in treating various medical conditions. Some of its scientific research applications include:
Chemistry: Studying the synthesis and reactions of amphetamine derivatives.
Biology: Investigating the effects of amphetamines on neurotransmitter release and receptor binding.
Medicine: Exploring the use of amphetamines in treating attention deficit hyperactivity disorder (ADHD) and narcolepsy.
Industry: Development of pharmaceutical formulations for weight loss and cognitive enhancement.
Mechanism of Action
Obetrol exerts its effects by increasing the release of neurotransmitters such as dopamine, norepinephrine, and serotonin in the brain. This leads to increased stimulation of the central nervous system, resulting in heightened alertness, reduced appetite, and increased energy levels. The molecular targets include dopamine and norepinephrine transporters, which are inhibited by amphetamines, leading to increased levels of these neurotransmitters in the synaptic cleft .
Comparison with Similar Compounds
Obetrol is similar to other amphetamine-based compounds, but it is unique in its combination of multiple amphetamine salts. Some similar compounds include:
Adderall: A reformulated version of Obetrol containing dextroamphetamine and racemic amphetamine salts.
Dexedrine: Contains dextroamphetamine sulfate.
Methamphetamine: Contains methamphetamine hydrochloride.
Phentermine: An amphetamine derivative used for weight loss.
Obetrol’s uniqueness lies in its specific combination of amphetamine salts, which was designed to provide a balanced and sustained release of the active ingredients .
Properties
IUPAC Name |
2,3,4,5-tetrahydroxyhexanedioic acid | |
---|---|---|
Details | Computed by Lexichem TK 2.7.0 (PubChem release 2021.05.07) | |
Source | PubChem | |
URL | https://pubchem.ncbi.nlm.nih.gov | |
Description | Data deposited in or computed by PubChem | |
InChI |
InChI=1S/C6H10O8/c7-1(3(9)5(11)12)2(8)4(10)6(13)14/h1-4,7-10H,(H,11,12)(H,13,14) | |
Details | Computed by InChI 1.0.6 (PubChem release 2021.05.07) | |
Source | PubChem | |
URL | https://pubchem.ncbi.nlm.nih.gov | |
Description | Data deposited in or computed by PubChem | |
InChI Key |
DSLZVSRJTYRBFB-UHFFFAOYSA-N | |
Details | Computed by InChI 1.0.6 (PubChem release 2021.05.07) | |
Source | PubChem | |
URL | https://pubchem.ncbi.nlm.nih.gov | |
Description | Data deposited in or computed by PubChem | |
Canonical SMILES |
C(C(C(C(=O)O)O)O)(C(C(=O)O)O)O | |
Details | Computed by OEChem 2.3.0 (PubChem release 2021.05.07) | |
Source | PubChem | |
URL | https://pubchem.ncbi.nlm.nih.gov | |
Description | Data deposited in or computed by PubChem | |
Molecular Formula |
C6H10O8 | |
Details | Computed by PubChem 2.1 (PubChem release 2021.05.07) | |
Source | PubChem | |
URL | https://pubchem.ncbi.nlm.nih.gov | |
Description | Data deposited in or computed by PubChem | |
Molecular Weight |
210.14 g/mol | |
Details | Computed by PubChem 2.1 (PubChem release 2021.05.07) | |
Source | PubChem | |
URL | https://pubchem.ncbi.nlm.nih.gov | |
Description | Data deposited in or computed by PubChem | |
CAS No. |
526-99-8 | |
Record name | mucic acid | |
Source | DTP/NCI | |
URL | https://dtp.cancer.gov/dtpstandard/servlet/dwindex?searchtype=NSC&outputformat=html&searchlist=8127 | |
Description | The NCI Development Therapeutics Program (DTP) provides services and resources to the academic and private-sector research communities worldwide to facilitate the discovery and development of new cancer therapeutic agents. | |
Explanation | Unless otherwise indicated, all text within NCI products is free of copyright and may be reused without our permission. Credit the National Cancer Institute as the source. | |
Synthesis routes and methods
Procedure details
Retrosynthesis Analysis
AI-Powered Synthesis Planning: Our tool employs the Template_relevance Pistachio, Template_relevance Bkms_metabolic, Template_relevance Pistachio_ringbreaker, Template_relevance Reaxys, Template_relevance Reaxys_biocatalysis model, leveraging a vast database of chemical reactions to predict feasible synthetic routes.
One-Step Synthesis Focus: Specifically designed for one-step synthesis, it provides concise and direct routes for your target compounds, streamlining the synthesis process.
Accurate Predictions: Utilizing the extensive PISTACHIO, BKMS_METABOLIC, PISTACHIO_RINGBREAKER, REAXYS, REAXYS_BIOCATALYSIS database, our tool offers high-accuracy predictions, reflecting the latest in chemical research and data.
Strategy Settings
Precursor scoring | Relevance Heuristic |
---|---|
Min. plausibility | 0.01 |
Model | Template_relevance |
Template Set | Pistachio/Bkms_metabolic/Pistachio_ringbreaker/Reaxys/Reaxys_biocatalysis |
Top-N result to add to graph | 6 |
Feasible Synthetic Routes
Disclaimer and Information on In-Vitro Research Products
Please be aware that all articles and product information presented on BenchChem are intended solely for informational purposes. The products available for purchase on BenchChem are specifically designed for in-vitro studies, which are conducted outside of living organisms. In-vitro studies, derived from the Latin term "in glass," involve experiments performed in controlled laboratory settings using cells or tissues. It is important to note that these products are not categorized as medicines or drugs, and they have not received approval from the FDA for the prevention, treatment, or cure of any medical condition, ailment, or disease. We must emphasize that any form of bodily introduction of these products into humans or animals is strictly prohibited by law. It is essential to adhere to these guidelines to ensure compliance with legal and ethical standards in research and experimentation.