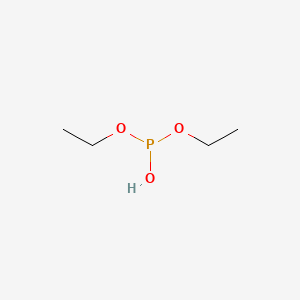
Ethyl phosphite, (C2H5O)2(OH)P
Overview
Description
Ethyl phosphite, (C2H5O)2(OH)P, is an organophosphorus compound with the molecular formula C4H11O3PThis compound is a colorless liquid with a boiling point of 187°C and a density of 1.072 g/mL . Ethyl phosphite is used in various chemical reactions and has applications in different fields, including chemistry, biology, and industry.
Preparation Methods
Ethyl phosphite can be synthesized through several methods. One common synthetic route involves the reaction of phosphorus trichloride with ethanol in the presence of a base, such as pyridine. The reaction proceeds as follows:
PCl3+3C2H5OH→(C2H5O)2(OH)P+2HCl
The reaction is typically carried out under reflux conditions, and the product is purified by distillation. Industrial production methods may involve similar reactions but on a larger scale, with optimized conditions to maximize yield and purity.
Chemical Reactions Analysis
Ethyl phosphite undergoes various chemical reactions, including oxidation, reduction, and substitution reactions. Some common reactions include:
-
Oxidation: : Ethyl phosphite can be oxidized to diethyl phosphate using oxidizing agents such as hydrogen peroxide or potassium permanganate.
(C2H5O)2(OH)P+H2O2→(C2H5O)2(O)P+H2O
-
Reduction: : Ethyl phosphite can be reduced to phosphorous acid using reducing agents like lithium aluminum hydride.
(C2H5O)2(OH)P+LiAlH4→H3PO3+2C2H5OH
-
Substitution: : Ethyl phosphite can undergo substitution reactions with halides to form various phosphonate esters.
(C2H5O)2(OH)P+RCl→(C2H5O)2(R)P+HCl
These reactions are typically carried out under controlled conditions to ensure the desired products are obtained .
Scientific Research Applications
Ethyl phosphite has several scientific research applications:
-
Chemistry: : It is used as a reagent in the synthesis of various organophosphorus compounds, including phosphonates and phosphates, which have applications in organic synthesis and catalysis .
-
Biology: : Ethyl phosphite derivatives are studied for their potential biological activities, including antioxidant, anticancer, antibacterial, and antifungal properties .
-
Medicine: : Some derivatives of ethyl phosphite are investigated for their potential use in drug development, particularly as prodrugs that can release active pharmaceutical ingredients under specific conditions .
-
Industry: : Ethyl phosphite is used in the production of flame retardants, plasticizers, and stabilizers for polymers .
Mechanism of Action
The mechanism of action of ethyl phosphite and its derivatives depends on the specific application. In general, ethyl phosphite can act as a ligand, coordinating with metal ions to form complexes that can catalyze various chemical reactions. In biological systems, ethyl phosphite derivatives may interact with cellular targets, such as enzymes or receptors, to exert their effects. The exact molecular targets and pathways involved vary depending on the specific derivative and application.
Comparison with Similar Compounds
Ethyl phosphite is similar to other organophosphorus compounds, such as triethyl phosphite and diethyl phosphonate. it has unique properties that make it suitable for specific applications. For example:
-
Triethyl phosphite: : This compound has three ethoxy groups attached to the phosphorus atom, making it more hydrophobic and less reactive than ethyl phosphite. It is commonly used as a ligand in coordination chemistry and as a reagent in organic synthesis .
-
Diethyl phosphonate: : This compound has two ethoxy groups and one phosphonate group attached to the phosphorus atom. It is more stable and less reactive than ethyl phosphite, making it suitable for use as a flame retardant and plasticizer .
Ethyl phosphite’s unique combination of reactivity and stability makes it a versatile compound for various applications.
Properties
IUPAC Name |
diethyl hydrogen phosphite | |
---|---|---|
Details | Computed by Lexichem TK 2.7.0 (PubChem release 2021.05.07) | |
Source | PubChem | |
URL | https://pubchem.ncbi.nlm.nih.gov | |
Description | Data deposited in or computed by PubChem | |
InChI |
InChI=1S/C4H11O3P/c1-3-6-8(5)7-4-2/h5H,3-4H2,1-2H3 | |
Details | Computed by InChI 1.0.6 (PubChem release 2021.05.07) | |
Source | PubChem | |
URL | https://pubchem.ncbi.nlm.nih.gov | |
Description | Data deposited in or computed by PubChem | |
InChI Key |
SULWMEGSVQCTSK-UHFFFAOYSA-N | |
Details | Computed by InChI 1.0.6 (PubChem release 2021.05.07) | |
Source | PubChem | |
URL | https://pubchem.ncbi.nlm.nih.gov | |
Description | Data deposited in or computed by PubChem | |
Canonical SMILES |
CCOP(O)OCC | |
Details | Computed by OEChem 2.3.0 (PubChem release 2021.05.07) | |
Source | PubChem | |
URL | https://pubchem.ncbi.nlm.nih.gov | |
Description | Data deposited in or computed by PubChem | |
Molecular Formula |
C4H11O3P | |
Details | Computed by PubChem 2.1 (PubChem release 2021.05.07) | |
Source | PubChem | |
URL | https://pubchem.ncbi.nlm.nih.gov | |
Description | Data deposited in or computed by PubChem | |
DSSTOX Substance ID |
DTXSID9041861 | |
Record name | Diethyl phosphite | |
Source | EPA DSSTox | |
URL | https://comptox.epa.gov/dashboard/DTXSID9041861 | |
Description | DSSTox provides a high quality public chemistry resource for supporting improved predictive toxicology. | |
Molecular Weight |
138.10 g/mol | |
Details | Computed by PubChem 2.1 (PubChem release 2021.05.07) | |
Source | PubChem | |
URL | https://pubchem.ncbi.nlm.nih.gov | |
Description | Data deposited in or computed by PubChem | |
CAS No. |
123-22-8, 762-04-9 | |
Record name | NSC72726 | |
Source | DTP/NCI | |
URL | https://dtp.cancer.gov/dtpstandard/servlet/dwindex?searchtype=NSC&outputformat=html&searchlist=72726 | |
Description | The NCI Development Therapeutics Program (DTP) provides services and resources to the academic and private-sector research communities worldwide to facilitate the discovery and development of new cancer therapeutic agents. | |
Explanation | Unless otherwise indicated, all text within NCI products is free of copyright and may be reused without our permission. Credit the National Cancer Institute as the source. | |
Record name | NSC5273 | |
Source | DTP/NCI | |
URL | https://dtp.cancer.gov/dtpstandard/servlet/dwindex?searchtype=NSC&outputformat=html&searchlist=5273 | |
Description | The NCI Development Therapeutics Program (DTP) provides services and resources to the academic and private-sector research communities worldwide to facilitate the discovery and development of new cancer therapeutic agents. | |
Explanation | Unless otherwise indicated, all text within NCI products is free of copyright and may be reused without our permission. Credit the National Cancer Institute as the source. | |
Record name | Diethyl phosphite | |
Source | EPA DSSTox | |
URL | https://comptox.epa.gov/dashboard/DTXSID9041861 | |
Description | DSSTox provides a high quality public chemistry resource for supporting improved predictive toxicology. | |
Disclaimer and Information on In-Vitro Research Products
Please be aware that all articles and product information presented on BenchChem are intended solely for informational purposes. The products available for purchase on BenchChem are specifically designed for in-vitro studies, which are conducted outside of living organisms. In-vitro studies, derived from the Latin term "in glass," involve experiments performed in controlled laboratory settings using cells or tissues. It is important to note that these products are not categorized as medicines or drugs, and they have not received approval from the FDA for the prevention, treatment, or cure of any medical condition, ailment, or disease. We must emphasize that any form of bodily introduction of these products into humans or animals is strictly prohibited by law. It is essential to adhere to these guidelines to ensure compliance with legal and ethical standards in research and experimentation.