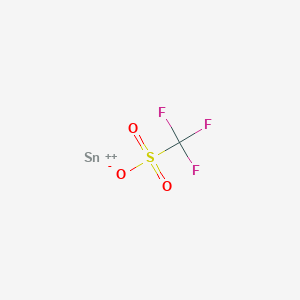
Tin(2+); trifluoromethanesulfonate
- Click on QUICK INQUIRY to receive a quote from our team of experts.
- With the quality product at a COMPETITIVE price, you can focus more on your research.
Overview
Description
Preparation Methods
Synthetic Routes and Reaction Conditions
Tin(2+); trifluoromethanesulfonate can be synthesized through the reaction of tin(2+) chloride with trifluoromethanesulfonic acid. The reaction typically occurs in an inert atmosphere to prevent oxidation and moisture contamination. The general reaction is as follows:
SnCl2+2CF3SO3H→(CF3SO3)2Sn+2HCl
The reaction mixture is then purified to obtain the desired product .
Industrial Production Methods
Industrial production of this compound follows a similar synthetic route but on a larger scale. The process involves careful control of reaction conditions, including temperature and pressure, to ensure high yield and purity. The product is then dried and packaged under an inert atmosphere to prevent degradation .
Chemical Reactions Analysis
Types of Reactions
Tin(2+); trifluoromethanesulfonate is known to undergo various types of reactions, including:
Aldol Reactions: It acts as a catalyst in stereoselective aldol reactions, facilitating the formation of carbon-carbon bonds.
Michael Reactions: It is used in Michael additions to form carbon-carbon bonds between a nucleophile and an α,β-unsaturated carbonyl compound.
Cycloaddition Reactions: It catalyzes [3+2] cycloaddition reactions, leading to the formation of five-membered rings.
Friedel-Crafts Reactions: It is employed in Friedel-Crafts acylation and alkylation reactions to introduce acyl or alkyl groups into aromatic compounds.
Common Reagents and Conditions
Common reagents used in reactions with this compound include aldehydes, ketones, enones, and aromatic compounds. The reactions are typically carried out under anhydrous conditions to prevent hydrolysis of the compound .
Major Products Formed
The major products formed from reactions involving this compound depend on the specific reaction type. For example:
Aldol Reactions: β-hydroxy carbonyl compounds
Michael Reactions: Michael adducts
Cycloaddition Reactions: Five-membered ring compounds
Friedel-Crafts Reactions: Acylated or alkylated aromatic compounds
Scientific Research Applications
Tin(2+); trifluoromethanesulfonate has a wide range of applications in scientific research, including:
Organic Synthesis: It is widely used as a catalyst in various organic synthesis reactions, including aldol, Michael, and Friedel-Crafts reactions.
Pharmaceuticals: It is employed in the synthesis of pharmaceutical intermediates and active pharmaceutical ingredients (APIs).
Polymer Chemistry: It is used in the polymerization of certain monomers to produce specialized polymers with unique properties.
Mechanism of Action
The mechanism of action of tin(2+); trifluoromethanesulfonate involves its role as a Lewis acid. It coordinates with electron-rich species, such as carbonyl groups, to activate them towards nucleophilic attack. This activation facilitates various organic reactions, including aldol and Michael reactions . The compound’s ability to stabilize reaction intermediates and transition states is crucial for its catalytic activity .
Comparison with Similar Compounds
Similar Compounds
- Tin(2+) chloride
- Tin(2+) iodide
- Tin(2+) fluoride
- Zinc trifluoromethanesulfonate
- Copper(2+) trifluoromethanesulfonate
Uniqueness
Tin(2+); trifluoromethanesulfonate is unique due to its high Lewis acidity and stability under anhydrous conditions. Unlike other tin(2+) compounds, it is highly soluble in organic solvents, making it suitable for a wide range of organic synthesis reactions . Its trifluoromethanesulfonate groups also provide additional stability and reactivity compared to other halide or pseudohalide tin(2+) compounds .
Properties
IUPAC Name |
tin(2+);trifluoromethanesulfonate |
Source
|
---|---|---|
Details | Computed by LexiChem 2.6.6 (PubChem release 2019.06.18) | |
Source | PubChem | |
URL | https://pubchem.ncbi.nlm.nih.gov | |
Description | Data deposited in or computed by PubChem | |
InChI |
InChI=1S/CHF3O3S.Sn/c2-1(3,4)8(5,6)7;/h(H,5,6,7);/q;+2/p-1 |
Source
|
Details | Computed by InChI 1.0.5 (PubChem release 2019.06.18) | |
Source | PubChem | |
URL | https://pubchem.ncbi.nlm.nih.gov | |
Description | Data deposited in or computed by PubChem | |
InChI Key |
IZICSWRWPHAWLH-UHFFFAOYSA-M |
Source
|
Details | Computed by InChI 1.0.5 (PubChem release 2019.06.18) | |
Source | PubChem | |
URL | https://pubchem.ncbi.nlm.nih.gov | |
Description | Data deposited in or computed by PubChem | |
Canonical SMILES |
C(F)(F)(F)S(=O)(=O)[O-].[Sn+2] |
Source
|
Details | Computed by OEChem 2.1.5 (PubChem release 2019.06.18) | |
Source | PubChem | |
URL | https://pubchem.ncbi.nlm.nih.gov | |
Description | Data deposited in or computed by PubChem | |
Molecular Formula |
CF3O3SSn+ |
Source
|
Details | Computed by PubChem 2.1 (PubChem release 2019.06.18) | |
Source | PubChem | |
URL | https://pubchem.ncbi.nlm.nih.gov | |
Description | Data deposited in or computed by PubChem | |
Molecular Weight |
267.78 g/mol |
Source
|
Details | Computed by PubChem 2.1 (PubChem release 2021.05.07) | |
Source | PubChem | |
URL | https://pubchem.ncbi.nlm.nih.gov | |
Description | Data deposited in or computed by PubChem | |
Disclaimer and Information on In-Vitro Research Products
Please be aware that all articles and product information presented on BenchChem are intended solely for informational purposes. The products available for purchase on BenchChem are specifically designed for in-vitro studies, which are conducted outside of living organisms. In-vitro studies, derived from the Latin term "in glass," involve experiments performed in controlled laboratory settings using cells or tissues. It is important to note that these products are not categorized as medicines or drugs, and they have not received approval from the FDA for the prevention, treatment, or cure of any medical condition, ailment, or disease. We must emphasize that any form of bodily introduction of these products into humans or animals is strictly prohibited by law. It is essential to adhere to these guidelines to ensure compliance with legal and ethical standards in research and experimentation.