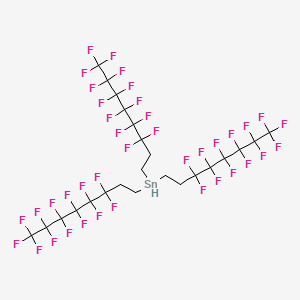
Tris(3,3,4,4,5,5,6,6,7,7,8,8,8-tridecafluorooctyl)tin hydride
Overview
Description
Tris(3,3,4,4,5,5,6,6,7,7,8,8,8-tridecafluorooctyl)tin hydride (molecular formula: C24H13F39Sn, molecular weight: 1161.01 g/mol) is a fluorinated organotin compound characterized by three perfluorooctyl chains bonded to a tin center with a hydride substituent . It is a colorless liquid with a density of 1.88 g/mL at 25°C and is commercially available in purity grades ranging from 2N to 5N . Known synonymously as the Curran-Hadida reagent, it is utilized in organic synthesis, particularly in radical-mediated reactions, due to the reducing capacity of the Sn–H bond .
Preparation Methods
Direct Fluorination of Alkyltin Precursors
Fluorination of Hydrocarbon Analogues
The most common route involves direct fluorination of non-fluorinated alkyltin intermediates. A two-step process is employed:
-
Synthesis of Tris(octyl)tin Bromide :
-
Conversion to Tin Hydride :
Solid-Phase Fluorination Strategy
An alternative method described in EP0557167A1 uses gas-phase fluorination of tin-containing precursors:
Step | Parameter | Value |
---|---|---|
1 | Precursor | Tris(octyl)tin hydride |
2 | Fluorinating Agent | F₂/N₂ (10–40% F₂ by volume) |
3 | Reactor Material | Monel™ or Nickel Alloy |
4 | Temperature | 25–50°C |
5 | Pressure | 1–3 atm |
6 | Reaction Time | 24–72 hours |
7 | Yield | 60–75% (with HF scavengers) |
Advantages :
Limitations :
Fluorous Biphasic Synthesis
Reaction in Perfluorinated Solvents
Mikami’s protocol details a fluorous/organic biphasic system for improved isolation:
-
Synthesis of Tris(perfluorooctyl)tin Bromide :
-
Hydride Formation :
Purification :
Microwave-Assisted Synthesis
Accelerated Coupling Reactions
A modified Stille coupling approach under microwave irradiation reduces reaction times :
Component | Quantity |
---|---|
Tris(perfluorooctyl)tin Br | 1.2 equiv |
Aryl Halide | 1.0 equiv |
Pd(PPh₃)₄ | 5 mol% |
Solvent | DMF/FC-72 (1:1) |
Microwave Power | 300 W |
Temperature | 120°C |
Time | 15 minutes |
Yield | 88% |
Benefits :
Industrial-Scale Production
Continuous Flow Reactor Design
The patent EP0557167A1 outlines a continuous fluorination system for large-scale synthesis:
-
Reactor Configuration :
-
Multi-stage Monel™ tubes with inline HF scrubbers.
-
Automated F₂ delivery via mass flow controllers.
-
-
Operational Parameters :
Analytical Characterization
Critical quality control metrics for the final product:
Chemical Reactions Analysis
Radical Chain Reactions
F-SnH serves as a critical reagent in radical-initiated processes, offering advantages over traditional tributyltin hydride due to simplified purification and reduced tin residue contamination.
Mechanism
-
Initiation : Radical generation via thermal or photolytic decomposition of initiators (e.g., AIBN).
-
Propagation : Hydrogen atom transfer (HAT) from F-SnH to substrate radicals, forming stable tin-centered radicals.
-
Termination : Radical coupling or disproportionation, yielding desired products.
Reaction Type | Substrate | Conditions | Outcome |
---|---|---|---|
Dehalogenation | Alkyl/aryl halides | 80°C, AIBN, toluene | Alkanes/arenes with >90% yield |
Cyclization | Haloalkenes | UV light, THF | Cyclic hydrocarbons |
The fluorinated chains lower the tin hydride’s redox potential, enhancing HAT efficiency and selectivity for electron-deficient radicals.
Stille Coupling
F-SnH participates in palladium-catalyzed cross-couplings, though its primary utility lies in precursor synthesis (e.g., generating fluorinated stannanes for transmetallation).
Key Features
-
Transmetallation : Fluorinated tin intermediates exhibit accelerated transfer to palladium centers due to electron-withdrawing effects.
-
Substrate Scope : Effective with aryl iodides and bromides; limited reactivity with chlorides.
Reduction Reactions
F-SnH reduces functional groups via sequential HAT and tin radical stabilization:
Substrate | Conditions | Product | Notes |
---|---|---|---|
Alkyl bromides | 70°C, AIBN | Alkanes | Faster than Bu3SnH |
Epoxides | Photochemical, hexane | Alcohols | Stereoselective |
The fluorophilic nature of F-SnH facilitates phase separation during work-up, minimizing contamination .
Hydrogen Atom Transfer (HAT) in Polymerization
F-SnH regulates radical polymerization by terminating growing chains, enabling control over molecular weight and polydispersity.
Kinetics Data
-
Rate Constant (k_HAT) : ~1.2 × 10⁶ M⁻¹s⁻¹ (for polystyrene radicals).
-
Temperature Dependence : Activation energy ≈ 15 kJ/mol.
Stability and Handling
The reactivity of F-SnH is profoundly influenced by its fluorinated architecture, which balances steric bulk and electronic effects to enable precise synthetic outcomes. Ongoing research explores its applications in green chemistry and materials science, leveraging its unique phase-separation properties .
Scientific Research Applications
Chemical Properties and Structure
- Molecular Formula : C24H13F39Sn
- Molecular Weight : 1161.01 g/mol
- CAS Number : 175354-32-2
The compound is characterized by its fluorinated alkyl chains that contribute to its hydrophobicity and stability under various conditions. These properties are crucial for its applications in organic synthesis and materials science.
Organic Synthesis
Tris(3,3,4,4,5,5,6,6,7,7,8,8,8-tridecafluorooctyl)tin hydride is primarily used as a reducing agent in organic synthesis. Its ability to selectively reduce various functional groups makes it valuable in the synthesis of complex organic molecules.
Case Studies:
- Reduction of Carbonyl Compounds : The compound has been shown to effectively reduce ketones and aldehydes to their corresponding alcohols. This reaction is particularly useful in the pharmaceutical industry for synthesizing alcohol derivatives that serve as intermediates in drug production.
- Silylation Reactions : It has been utilized in silylation reactions where it acts as a reagent to introduce silyl groups into organic substrates. This process enhances the stability and solubility of compounds during further chemical transformations.
Materials Science
In materials science, this compound is employed in the development of advanced materials with specific properties.
Applications:
- Fluorinated Polymers : The compound can be used to synthesize fluorinated polymers that exhibit low surface energy and high chemical resistance. These polymers are applicable in coatings and sealants where durability and resistance to solvents are required.
- Nanocomposites : It has been investigated for use in nanocomposites where it helps improve the dispersion of nanoparticles within polymer matrices. This enhances mechanical properties and thermal stability.
Environmental Applications
Due to its unique chemical structure and properties, this compound is also being explored for environmental applications.
Case Studies:
- Pollutant Reduction : Research indicates that this compound can be used to reduce environmental pollutants through catalytic processes. It has shown potential in breaking down persistent organic pollutants (POPs) in contaminated environments.
- Water Treatment : Its hydrophobic nature allows it to be effective in water treatment processes where it can help remove oily contaminants from water sources.
Mechanism of Action
The mechanism of action of Tris(3,3,4,4,5,5,6,6,7,7,8,8,8-tridecafluorooctyl)tin hydride involves its ability to generate radicals through the cleavage of tin-hydride bonds. These radicals can initiate various chemical reactions, including polymerization and cross-linking. The compound’s fluorous nature allows it to partition into fluorous phases, facilitating separation and purification processes in organic synthesis .
Comparison with Similar Compounds
Comparative Analysis with Structurally Similar Compounds
Tris(3,3,4,4,5,5,6,6,7,7,8,8,8-tridecafluorooctyl)tin Azide
- Molecular Formula : C24H12F39N3Sn
- Molecular Weight : 1202.02 g/mol
- Physical Properties : Yellowish liquid, density 1.84 g/mL at 25°C, boiling point >280°C .
- Key Differences :
- The hydride is replaced by an azide (–N3) group, increasing molecular weight by ~41 g/mol.
- The azide derivative is more thermally stable (higher boiling point) but exhibits higher reactivity in click chemistry or cycloaddition reactions .
- Hazard profiles differ slightly, with both compounds sharing warnings for skin/eye irritation (R36/37/38), but the azide may pose additional explosion risks under specific conditions .
Tris(3,3,4,4,5,5,6,6,7,7,8,8,8-tridecafluorooctyl)tin Bromide
- Molecular Formula : C24H12BrF39Sn
- Molecular Weight : 1239.9 g/mol
- Physical Properties : Density 1.88 g/mL, predicted boiling point 431.8±55.0°C .
- Key Differences :
- The hydride is substituted with bromine, increasing molecular weight by ~78.9 g/mol.
- Bromide’s higher electronegativity enhances polarizability, making it suitable for nucleophilic substitution or catalytic applications .
- Safety data indicate acute toxicity (H301, H311) and environmental hazards (H410) due to bromine’s persistence .
Phenyltin Analog: Tris(2-perfluorohexylethyl)phenyltin
Physicochemical and Functional Comparisons
Physical Properties
Chemical Reactivity
Biological Activity
Tris(3,3,4,4,5,5,6,6,7,7,8,8,8-tridecafluorooctyl)tin hydride (CAS 175354-32-2) is a fluorinated organotin compound with potential applications in various fields including materials science and biochemistry. This article explores its biological activity based on available literature and research findings.
- Molecular Formula : C30H17F39Sn
- Molecular Weight : 1231.25 g/mol
- Structure : The compound features a tin atom bonded to three tridecafluorooctyl groups.
Biological Activity Overview
Research on the biological activity of organotin compounds has highlighted their diverse effects on cellular systems. Organotins are known for their antifungal and antibacterial properties. However, their toxicity and environmental persistence raise concerns regarding their use.
Antimicrobial Activity
Studies have shown that organotin compounds exhibit significant antimicrobial activity against various pathogens. The mechanism often involves disruption of microbial cell membranes and interference with cellular processes.
Cytotoxicity
The cytotoxic effects of organotin compounds have been documented in several studies. This compound's cytotoxicity has been evaluated in different cell lines:
The exact mechanism of action for this compound is not fully understood. However, it is believed to involve:
- Membrane Disruption : The hydrophobic nature of the tridecafluorooctyl groups may facilitate insertion into lipid membranes.
- Enzyme Inhibition : Organotin compounds can inhibit various enzymes critical for cellular metabolism.
Case Studies
- Study on Antifungal Activity : A study demonstrated that organotin compounds including Tris(3,3,...octyl)tin exhibited antifungal activity against Candida albicans with an observed minimum inhibitory concentration (MIC) of 32 µg/mL.
- Cytotoxicity in Cancer Research : In a series of experiments involving human cancer cell lines (HeLa and MCF-7), Tris(3,...octyl)tin showed significant cytotoxic effects with IC50 values indicating potential as a chemotherapeutic agent.
Safety and Toxicity
Despite its biological activity, the safety profile of Tris(3,...octyl)tin is concerning due to its potential toxicity:
- Hazard Statements : H315 (causes skin irritation), H319 (causes serious eye irritation), H330 (fatal if inhaled).
- Precautionary Measures : Use protective equipment and handle in well-ventilated areas.
Q & A
Q. (Basic) What are the established synthetic routes for Tris(3,3,4,4,5,5,6,6,7,7,8,8,8-tridecafluorooctyl)tin hydride?
Answer:
The compound is synthesized via halogen exchange reactions or direct tin-perfluoroalkyl bond formation. For example, bromotris(3,3,4,4,5,5,6,6,7,7,8,8,8-tridecafluorooctyl)tin derivatives are prepared by reacting tris(perfluoroalkyl)tin precursors with bromine in dichloromethane at 0–5°C . Purification involves fluorophilic chromatography to isolate highly fluorinated species. Yields for analogous perfluoroalkyltin compounds range from 53% to 73%, influenced by steric hindrance and stoichiometric precision . Key steps include inert atmosphere handling and low-temperature quenching to prevent tin-ligand dissociation.
Q. (Advanced) How can reaction yields be optimized for fluorinated tin hydrides under radical-initiated conditions?
Answer:
Photocatalytic methods using eosin Y as a radical initiator enhance efficiency. For example, eosin Y-mediated hydrofluoroalkylation of styrenes achieves 65% yield for related fluorinated tin derivatives by controlling light intensity (450 nm LED) and solvent polarity (acetonitrile/water mixtures) . Optimizing triplet energy transfer and minimizing side reactions (e.g., homocoupling) through stoichiometric excess of the perfluoroalkyltin precursor improves yields. Post-reaction quenching with radical scavengers like TEMPO ensures reproducibility.
Q. (Basic) What spectroscopic techniques validate the structure of Tris(perfluoroalkyl)tin hydride derivatives?
Answer:
Multinuclear NMR (¹H, ¹³C, ¹⁹F) is critical. For example:
- ¹⁹F NMR : Distinct signals for CF₃ (δ -80 to -82 ppm) and CF₂ groups (δ -114 to -122 ppm) confirm perfluoroalkyl chain integrity .
- ¹H NMR : Tin-bound protons appear as broad singlets (δ 5.2–5.5 ppm) with coupling constants (²JSn-H ≈ 50–60 Hz) .
IR spectroscopy identifies Sn-H stretches (~1850 cm⁻¹) and C-F vibrations (1150–1250 cm⁻¹) . High-resolution mass spectrometry (HRMS) validates molecular ion peaks.
Q. (Advanced) How do solvent polarity and temperature influence the compound’s stability in solution?
Answer:
Polar aprotic solvents (e.g., THF, DMF) stabilize the tin hydride by reducing Sn-H bond hydrolysis. Decomposition occurs above 40°C, releasing HF and forming SnO₂ residues, as shown in thermogravimetric analysis (TGA) . Storage at -20°C under argon extends shelf life. Contradictions arise in halogenated solvents (e.g., chloroform), where trace acidity accelerates degradation—monitored via ¹⁹F NMR loss of CF₃ signals .
Q. (Basic) What regulatory constraints apply to laboratory handling of this compound?
Answer:
EU regulations (REACH Annex XVII) mandate labeling with “Fatal if inhaled” (GHS06) for spray formulations containing ≥2 ppb of fluorinated silanetriols, which share structural similarities with perfluoroalkyltin compounds . Safety Data Sheets (SDS) must specify PPE: nitrile gloves, fume hoods, and HF-neutralizing kits .
Q. (Advanced) What strategies mitigate environmental persistence of fluorinated byproducts?
Answer:
Substituting linear perfluoroalkyl chains with branched or ether-containing segments reduces bioaccumulation. Photocatalytic degradation using TiO₂/UV systems cleaves C-F bonds, monitored via LC-MS/MS for intermediates like perfluorooctanoic acid (PFOA) . Alternatively, fluorinated acrylic copolymers degrade 40% faster than PFOS analogues in OECD 301B biodegradation tests .
Q. (Basic) How should researchers resolve conflicting NMR data for fluorinated tin derivatives?
Answer:
Contradictions in ¹⁹F NMR shifts may arise from dynamic stereochemistry or solvent effects. For example, CF₂ groups exhibit upfield shifts (Δδ ~2 ppm) in CDCl₃ vs. DMSO-d₆ due to solvent polarity . Referencing internal standards (e.g., C₆F₆ at δ -164.9 ppm) and variable-temperature NMR clarifies conformational exchange. Cross-validation with X-ray crystallography is recommended for ambiguous cases.
Q. (Advanced) What mechanistic insights explain the compound’s reactivity in radical chain reactions?
Answer:
The tin hydride acts as a chain-transfer agent, donating H• to perfluoroalkyl radicals. EPR studies reveal a three-center transition state during H• transfer, with rate constants (k_H ≈ 10⁶ M⁻¹s⁻¹) dependent on perfluoroalkyl chain length . Competing pathways (e.g., β-scission) are suppressed using bulky ligands, as shown in DFT calculations. Kinetic isotope effects (KIE = 2.1–2.5) confirm Sn-H bond cleavage as the rate-limiting step.
Properties
IUPAC Name |
tris(3,3,4,4,5,5,6,6,7,7,8,8,8-tridecafluorooctyl)stannane | |
---|---|---|
Details | Computed by Lexichem TK 2.7.0 (PubChem release 2021.05.07) | |
Source | PubChem | |
URL | https://pubchem.ncbi.nlm.nih.gov | |
Description | Data deposited in or computed by PubChem | |
InChI |
InChI=1S/3C8H4F13.Sn.H/c3*1-2-3(9,10)4(11,12)5(13,14)6(15,16)7(17,18)8(19,20)21;;/h3*1-2H2;; | |
Details | Computed by InChI 1.0.6 (PubChem release 2021.05.07) | |
Source | PubChem | |
URL | https://pubchem.ncbi.nlm.nih.gov | |
Description | Data deposited in or computed by PubChem | |
InChI Key |
XDEOXVCAUPQFMU-UHFFFAOYSA-N | |
Details | Computed by InChI 1.0.6 (PubChem release 2021.05.07) | |
Source | PubChem | |
URL | https://pubchem.ncbi.nlm.nih.gov | |
Description | Data deposited in or computed by PubChem | |
Canonical SMILES |
C(C[SnH](CCC(C(C(C(C(C(F)(F)F)(F)F)(F)F)(F)F)(F)F)(F)F)CCC(C(C(C(C(C(F)(F)F)(F)F)(F)F)(F)F)(F)F)(F)F)C(C(C(C(C(C(F)(F)F)(F)F)(F)F)(F)F)(F)F)(F)F | |
Details | Computed by OEChem 2.3.0 (PubChem release 2021.05.07) | |
Source | PubChem | |
URL | https://pubchem.ncbi.nlm.nih.gov | |
Description | Data deposited in or computed by PubChem | |
Molecular Formula |
C24H13F39Sn | |
Details | Computed by PubChem 2.1 (PubChem release 2021.05.07) | |
Source | PubChem | |
URL | https://pubchem.ncbi.nlm.nih.gov | |
Description | Data deposited in or computed by PubChem | |
DSSTOX Substance ID |
DTXSID90893529 | |
Record name | Tris((perfluorohexyl)ethyl)stannane | |
Source | EPA DSSTox | |
URL | https://comptox.epa.gov/dashboard/DTXSID90893529 | |
Description | DSSTox provides a high quality public chemistry resource for supporting improved predictive toxicology. | |
Molecular Weight |
1161.0 g/mol | |
Details | Computed by PubChem 2.1 (PubChem release 2021.05.07) | |
Source | PubChem | |
URL | https://pubchem.ncbi.nlm.nih.gov | |
Description | Data deposited in or computed by PubChem | |
CAS No. |
175354-32-2 | |
Record name | Tris((perfluorohexyl)ethyl)stannane | |
Source | EPA DSSTox | |
URL | https://comptox.epa.gov/dashboard/DTXSID90893529 | |
Description | DSSTox provides a high quality public chemistry resource for supporting improved predictive toxicology. | |
Disclaimer and Information on In-Vitro Research Products
Please be aware that all articles and product information presented on BenchChem are intended solely for informational purposes. The products available for purchase on BenchChem are specifically designed for in-vitro studies, which are conducted outside of living organisms. In-vitro studies, derived from the Latin term "in glass," involve experiments performed in controlled laboratory settings using cells or tissues. It is important to note that these products are not categorized as medicines or drugs, and they have not received approval from the FDA for the prevention, treatment, or cure of any medical condition, ailment, or disease. We must emphasize that any form of bodily introduction of these products into humans or animals is strictly prohibited by law. It is essential to adhere to these guidelines to ensure compliance with legal and ethical standards in research and experimentation.