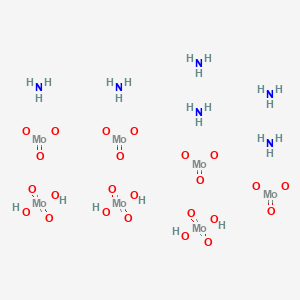
Ammonium paramolybdate
Overview
Description
Ammonium paramolybdate (APM), chemically denoted as (NH₄)₆Mo₇O₂₄·4H₂O, is a key intermediate in molybdenum metallurgy and chemical synthesis. It crystallizes as a tetrahydrate, featuring the heptamolybdate anion [Mo₇O₂₄]⁶⁻, which forms a complex layered structure with ammonium ions and water molecules . APM is industrially significant as a precursor to molybdenum trioxide (MoO₃) and molybdenum metal, both critical for catalysts, alloys, and electronic materials.
Production and Decomposition:
APM is synthesized via acidification of ammonium molybdate solutions. Upon heating, it undergoes stepwise decomposition, releasing NH₃ and H₂O, and forming intermediates such as (NH₄)₄Mo₅O₁₇ and (NH₄)₂Mo₄O₁₃ before yielding MoO₃ . This thermal behavior is exploited in catalyst preparation, where controlled calcination tailors MoO₃’s surface properties for applications in hydrodesulfurization and selective oxidation reactions .
Preparation Methods
Ammonium paramolybdate can be synthesized by dissolving molybdenum trioxide (MoO₃) in an excess of aqueous ammonia (NH₃) and then evaporating the solution at room temperature. As the solution evaporates, the excess ammonia escapes, resulting in the formation of six-sided transparent prisms of the tetrahydrate form of this compound . Industrially, this compound is produced as an intermediate product in the hydrometallurgical process of molybdenum using ammonia solution .
Chemical Reactions Analysis
Thermal Decomposition
Upon heating, ammonium paramolybdate undergoes stepwise decomposition, releasing NH₃ and H₂O, ultimately forming MoO₃. The pathway is temperature-dependent:
Step | Temperature Range | Reaction | Product |
---|---|---|---|
1 | 90–100°C | (NH₄)₆Mo₇O₂₄·4H₂O → (NH₄)₄Mo₅O₁₇ + 2NH₃ + 21H₂O | Anhydrous ammonium molybdate |
2 | 190–575°C | (NH₄)₄Mo₅O₁₇ → 5MoO₃ + 4NH₃ + 2H₂O | Molybdenum trioxide (MoO₃) |
3 | >575°C | MoO₃ + NH₃ → MoO₂ + N₂ + H₂O (self-reduction via residual ammonia) | Molybdenum dioxide (MoO₂) |
At 575°C, residual ammonia in intermediate phases like (NH₄)₄Mo₅O₁₇ facilitates partial self-reduction, achieving up to 18% conversion to MoO₂ without external reductants .
Reaction with Acids
In acidic environments, this compound reacts to form molybdic acid (H₂MoO₄) and ammonium salts:
text(NH₄)₆Mo₇O₂₄ + 14HCl → 7H₂MoO₄ + 6NH₄Cl + 4H₂O
This reaction is pivotal in analytical chemistry for detecting phosphates and arsenates, where molybdic acid forms colored complexes . The pH of concentrated solutions stabilizes between 5–6 .
Reduction Reactions
Under hydrogen flow, this compound derivatives reduce to molybdenum metal:
textMoO₃ + 3H₂ → Mo + 3H₂O (Two-stage reduction: MoO₃ → MoO₂ → Mo)
Direct reduction of (NH₄)₄Mo₅O₁₇ with H₂ at 575°C achieves 100% conversion to MoO₂ within 30 minutes, outperforming pure MoO₃ reduction rates (80% under similar conditions) .
Solid-Gas Phase Transformations
Recent studies demonstrate stoichiometric reactions between ammonium tetramolybdate ((NH₄)₂Mo₄O₁₃) and NH₄HCO₃ to yield paramolybdate:
text(NH₄)₂Mo₄O₁₃ + NH₄HCO₃ → (NH₄)₆Mo₇O₂₄·4H₂O + CO₂↑
This method avoids traditional sublimation routes, enhancing sustainability .
Key Research Findings
-
Morphology Changes : Thermal decomposition transforms crystalline paramolybdate into platelet-shaped MoO₂, critical for metallurgical applications .
-
Self-Reduction Efficiency : Residual ammonia in (NH₄)₄Mo₅O₁₇ reduces hydrogen consumption by 18% during MoO₂ production, optimizing industrial processes .
Scientific Research Applications
Analytical Chemistry
Role as an Analytical Reagent
Ammonium paramolybdate is widely used as an analytical reagent for detecting and quantifying phosphates, silicates, arsenates, and lead in aqueous solutions. This capability is crucial in environmental monitoring, particularly in assessing water quality in rivers and seas .
Table 1: Analytical Applications of this compound
Application | Description | Importance |
---|---|---|
Phosphate Detection | Forms a colored complex with phosphates | Essential for water quality analysis |
Silicate Measurement | Used in silicate analysis through colorimetric methods | Vital for geological and environmental studies |
Arsenate Detection | Confirmatory test for arsenic in water samples | Important for public health monitoring |
Environmental Applications
Photocatalysis and Water Purification
this compound has been investigated for its photocatalytic properties, particularly in the degradation of organic pollutants in water. It is often combined with other materials like titanium dioxide (TiO2) to enhance photocatalytic efficiency .
Case Study: Photocatalytic Water Treatment
A study demonstrated that composites of this compound with TiO2 significantly improved the degradation rates of common pollutants under UV light exposure. The results indicated a reduction of contaminants by over 90% within a few hours of treatment, showcasing its potential for practical applications in wastewater treatment .
Industrial Applications
Catalyst in Chemical Reactions
In industrial settings, this compound serves as a catalyst for various reactions, including desulfurization processes in petroleum refining and the production of molybdenum metal . Its role as a catalyst is particularly important due to its ability to facilitate reactions at lower temperatures while maintaining high efficiency.
Table 2: Industrial Uses of this compound
Application | Description | Industry |
---|---|---|
Desulfurization | Catalyzes the removal of sulfur from hydrocarbons | Petroleum refining |
Molybdenum Production | Key intermediate for producing molybdenum metal | Metallurgy |
Electroplating | Used in electroplating processes | Electronics and manufacturing |
Medical Applications
Use in Toxicology Testing
this compound has been utilized in toxicology, particularly as a confirmatory test for aluminum phosphide poisoning. It reacts with phosphine gas released during aluminum phosphide decomposition, aiding in the diagnosis of poisoning cases .
Case Study: Aluminum Phosphide Poisoning
In clinical settings, this compound was employed to confirm cases of aluminum phosphide poisoning through a specific colorimetric reaction. This application highlights its importance not only in analytical chemistry but also in medical diagnostics .
Research Innovations
Recent studies have explored the thermal decomposition of this compound to produce molybdenum oxides, which are valuable in various applications such as catalysis and materials science. The self-reduction properties of ammonium molybdates during thermal treatment have been investigated to optimize the production of molybdenum metal from its compounds .
Table 3: Research Innovations Involving this compound
Research Focus | Findings | Implications |
---|---|---|
Thermal Decomposition | Produces MoO3 and reduces reaction time | Enhances efficiency in molybdenum production |
Self-Reduction Properties | Residual ammonia acts as a reductant | Potentially lowers costs and improves yields |
Mechanism of Action
The mechanism of action of ammonium paramolybdate involves its role as a source of molybdenum, which is an essential element in various enzymes. Molybdenum is present in enzymes such as xanthine oxidase, sulfite oxidase, and aldehyde oxidase, where it acts as a cofactor and plays a crucial role in the catalytic activity of these enzymes . These enzymes are involved in important metabolic pathways, including the oxidation of purines, the detoxification of sulfites, and the metabolism of aldehydes.
Comparison with Similar Compounds
Comparison with Similar Compounds
Ammonium Molybdate ((NH₄)₂MoO₄)
Chemical Structure : A simpler molybdate with a 1:1 Mo:NH₄⁺ ratio, lacking the polynuclear structure of APM .
Thermal Behavior : Decomposes directly to MoO₃ without forming intermediate polymolybdates, as it lacks the structural complexity of APM .
Ammonium Polymolybdates (e.g., (NH₄)₄Mo₅O₁₇)
Chemical Structure : Intermediate phases formed during APM decomposition, with varying Mo:NH₄⁺ ratios (e.g., (NH₄)₄Mo₅O₁₇ has a 5:4 ratio) .
Thermal Behavior :
- Exhibit stepwise decomposition akin to APM but with distinct intermediates.
- (NH₄)₄Mo₅O₁₇ converts to MoO₂ under hydrogen reduction, a pathway leveraged in MoO₂ synthesis for battery electrodes .
Potassium Heptamolybdate (K₆[Mo₇O₂₄]·4H₂O)
Chemical Structure : Isostructural to APM, with K⁺ replacing NH₄⁺ in the lattice .
Thermal Behavior : Decomposes similarly to APM but requires higher temperatures due to potassium’s thermal stability.
MoO₂(DDTC)₂ Complex
Chemical Structure : A molybdenum-dithiocarbamate complex synthesized from APM and diethyl dithiocarbamate (DDTC) .
Thermal Behavior : Decomposes under hydrothermal conditions to form MoS₂, a catalyst for hydrodesulfurization .
Data Table: Comparative Properties
*Mo content calculated for MoO₂(DDTC)₂.
Research Findings and Industrial Relevance
- Catalyst Design : APM’s controlled decomposition enables MoO₃ with tunable acidity and surface area, critical for selective oxidation of isobutylene to methacrolein (87.2% conversion, 72% selectivity) .
- Environmental Applications : APM-derived MoS₂ suppresses polycondensation in heavy oil hydroconversion, enhancing yields of light fractions .
- Structural Insights : The heptamolybdate anion’s stability in APM and its potassium analog underscores its role in polynuclear molybdate chemistry .
Contradictions and Clarifications :
- mislabels (NH₄)₂MoO₄ as "this compound," a misnomer corrected in technical contexts .
Biological Activity
Ammonium paramolybdate, also known as ammonium heptamolybdate, is an inorganic compound with the formula . It is a significant source of molybdenum, an essential trace element in biological systems. This article explores the biological activity of this compound, including its roles in enzymatic functions, potential therapeutic applications, and its use in analytical chemistry.
This compound is typically encountered as a colorless solid. The compound crystallizes in a tetrahydrate form and has a pH range of 5 to 6 in concentrated solutions. Its structure consists of octahedral Mo centers coordinated by oxide ligands, which can be terminal or bridging .
Biological Importance of Molybdenum
Molybdenum plays a crucial role in various biological processes. It is a cofactor for several enzymes, including:
- Xanthine oxidase : Involved in purine metabolism.
- Sulfite oxidase : Essential for sulfur metabolism.
- Aldehyde oxidase : Participates in the oxidation of aldehydes.
Molybdenum facilitates oxygen transfer reactions and shuttles between oxidation states and , which are vital for enzymatic activity .
Therapeutic Applications
- Nutritional Supplementation : Molybdenum deficiency can lead to health issues such as kidney stones and other metabolic disorders. This compound is sometimes used in Total Parenteral Nutrition (TPN) to provide necessary molybdenum levels .
- Toxicology : The compound has been utilized in toxicological studies, particularly in diagnosing aluminum phosphide poisoning. The ammonium molybdate test serves as a confirmatory method for detecting phosphine gas released during aluminum phosphide decomposition .
Analytical Applications
This compound is widely employed as an analytical reagent due to its ability to form colored complexes with various anions. Its applications include:
- Phosphate Detection : It reacts with phosphates to form a blue complex, facilitating quantitative analysis in environmental samples .
- Negative Staining in Electron Microscopy : Used at concentrations of 3-5% for visualizing biological specimens under electron microscopy due to its electron-dense properties .
Case Study 1: Aluminum Phosphide Poisoning
A study highlighted the importance of ammonium molybdate in diagnosing aluminum phosphide poisoning. The test confirmed the presence of phosphine gas, which is critical for timely medical intervention .
Case Study 2: Enzymatic Activity
Research has shown that molybdenum-containing enzymes exhibit variable activity based on the availability of molybdenum sources like this compound. This has implications for understanding metabolic pathways and the impact of trace element availability on health .
Research Findings
Recent studies have focused on the thermal decomposition kinetics of this compound, revealing that it decomposes into various products at elevated temperatures, which can influence its biological activity and applications .
Data Table: Biological Roles of Molybdenum Enzymes
Enzyme | Function | Molybdenum Role |
---|---|---|
Xanthine Oxidase | Purine metabolism | Catalyzes oxidation |
Sulfite Oxidase | Sulfur metabolism | Electron transfer |
Aldehyde Oxidase | Aldehyde oxidation | Substrate interaction |
Q & A
Basic Questions
Q. What are the standard methods for synthesizing ammonium paramolybdate, and how can purity be verified?
this compound is typically synthesized by dissolving molybdenum trioxide (MoO₃) in aqueous ammonia under controlled pH (5–6). The solution is evaporated at ambient temperature to form crystalline products . Purity verification involves X-ray diffraction (XRD) to confirm crystallinity and spectroscopic techniques like Fourier-transform infrared (FTIR) to detect Mo-O bonding patterns .
Q. What spectroscopic techniques are recommended for identifying solute clustering in this compound solutions?
Laser Raman spectroscopy and multi-angle light scattering are primary methods. Prepare solutions across concentration gradients (undersaturated to supersaturated) and analyze Raman shifts for Mo-O-Mo bridging modes. Light scattering detects particle size changes indicative of clustering .
Q. How should researchers handle and store this compound to ensure safety and stability during experiments?
Store in airtight containers away from moisture and acids to prevent hydrolysis or decomposition. Use PPE (gloves, goggles) to avoid inhalation/contact, as it is a respiratory irritant. Stability tests under varying temperatures (20–100°C) can preempt degradation .
Q. What are the recommended procedures for determining phosphorus content using this compound-based reagents?
Use Olsen and Dean’s digestion method: Treat samples with perchloric acid (HClO₄) and mix with this compound-vanadate reagent. Measure absorbance at 400–420 nm via UV-Vis spectroscopy to quantify phosphorus via the molybdenum blue complex .
Advanced Research Questions
Q. How can the nuclear quadrupole interaction in this compound be characterized, and what insights does this provide into its structure?
Time-differential perturbed angular correlation (TDPAC) with ⁹⁹Tc isotopes probes Mo-oxygen octahedral distortions. This method identifies three distinct octahedral classes (population ratio 4:2:1), aligning with crystallographic models of edge/corner-sharing polyhedra .
Q. What experimental approaches resolve discrepancies in reported crystal structures of this compound?
Combine XRD with neutron diffraction to resolve hydrogen positions in NH₄⁺ groups. Pair with thermogravimetric analysis (TGA) to correlate dehydration/deammoniation steps (e.g., weight loss at 130–375°C) with structural transitions .
Q. How do pH and concentration gradients influence solute clustering in this compound solutions?
Design pH-controlled experiments (pH 2–10) using buffer systems. Monitor clustering via dynamic light scattering (DLS) at varying concentrations (0.1–2.0 M). Clustering increases in supersaturated solutions (pH <5) due to protonation of Mo-O bonds .
Q. What strategies optimize catalytic activity in molybdenum-based systems derived from this compound?
Pre-calcine this compound at 400–500°C to form MoO₃, then reduce in H₂/Ar to create MoOₓ defect sites. Characterize active sites using X-ray photoelectron spectroscopy (XPS) and quantify activity via turnover frequency (TOF) in test reactions (e.g., dehydrogenation) .
Q. How can isotopic labeling (e.g., ⁹⁹Tc) enhance structural dynamics studies of this compound-derived catalysts?
Substitute Mo with ⁹⁹Tc during synthesis to enable gamma-ray detection of quadrupole interactions. This reveals real-time structural changes during catalytic cycles, such as oxygen vacancy formation .
Q. What experimental setups analyze the thermal decomposition pathways of this compound?
Use coupled TGA-mass spectrometry to track NH₃ and H₂O release. Dehydration occurs at 130–280°C, followed by deammoniation at 360–375°C. Isotopic labeling (¹⁵N) in NH₄⁺ distinguishes between NH₃ and N₂ evolution .
Properties
IUPAC Name |
azane;dihydroxy(dioxo)molybdenum;trioxomolybdenum | |
---|---|---|
Details | Computed by LexiChem 2.6.6 (PubChem release 2019.06.18) | |
Source | PubChem | |
URL | https://pubchem.ncbi.nlm.nih.gov | |
Description | Data deposited in or computed by PubChem | |
InChI |
InChI=1S/7Mo.6H3N.6H2O.18O/h;;;;;;;6*1H3;6*1H2;;;;;;;;;;;;;;;;;;/q;;;;3*+2;;;;;;;;;;;;;;;;;;;;;;;;;;;;;;/p-6 | |
Details | Computed by InChI 1.0.5 (PubChem release 2019.06.18) | |
Source | PubChem | |
URL | https://pubchem.ncbi.nlm.nih.gov | |
Description | Data deposited in or computed by PubChem | |
InChI Key |
PTPRBYUXSYJORU-UHFFFAOYSA-H | |
Details | Computed by InChI 1.0.5 (PubChem release 2019.06.18) | |
Source | PubChem | |
URL | https://pubchem.ncbi.nlm.nih.gov | |
Description | Data deposited in or computed by PubChem | |
Canonical SMILES |
N.N.N.N.N.N.O[Mo](=O)(=O)O.O[Mo](=O)(=O)O.O[Mo](=O)(=O)O.O=[Mo](=O)=O.O=[Mo](=O)=O.O=[Mo](=O)=O.O=[Mo](=O)=O | |
Details | Computed by OEChem 2.1.5 (PubChem release 2019.06.18) | |
Source | PubChem | |
URL | https://pubchem.ncbi.nlm.nih.gov | |
Description | Data deposited in or computed by PubChem | |
Molecular Formula |
H24Mo7N6O24 | |
Details | Computed by PubChem 2.1 (PubChem release 2019.06.18) | |
Source | PubChem | |
URL | https://pubchem.ncbi.nlm.nih.gov | |
Description | Data deposited in or computed by PubChem | |
Molecular Weight |
1163.9 g/mol | |
Details | Computed by PubChem 2.1 (PubChem release 2021.05.07) | |
Source | PubChem | |
URL | https://pubchem.ncbi.nlm.nih.gov | |
Description | Data deposited in or computed by PubChem | |
Retrosynthesis Analysis
AI-Powered Synthesis Planning: Our tool employs the Template_relevance Pistachio, Template_relevance Bkms_metabolic, Template_relevance Pistachio_ringbreaker, Template_relevance Reaxys, Template_relevance Reaxys_biocatalysis model, leveraging a vast database of chemical reactions to predict feasible synthetic routes.
One-Step Synthesis Focus: Specifically designed for one-step synthesis, it provides concise and direct routes for your target compounds, streamlining the synthesis process.
Accurate Predictions: Utilizing the extensive PISTACHIO, BKMS_METABOLIC, PISTACHIO_RINGBREAKER, REAXYS, REAXYS_BIOCATALYSIS database, our tool offers high-accuracy predictions, reflecting the latest in chemical research and data.
Strategy Settings
Precursor scoring | Relevance Heuristic |
---|---|
Min. plausibility | 0.01 |
Model | Template_relevance |
Template Set | Pistachio/Bkms_metabolic/Pistachio_ringbreaker/Reaxys/Reaxys_biocatalysis |
Top-N result to add to graph | 6 |
Feasible Synthetic Routes
Disclaimer and Information on In-Vitro Research Products
Please be aware that all articles and product information presented on BenchChem are intended solely for informational purposes. The products available for purchase on BenchChem are specifically designed for in-vitro studies, which are conducted outside of living organisms. In-vitro studies, derived from the Latin term "in glass," involve experiments performed in controlled laboratory settings using cells or tissues. It is important to note that these products are not categorized as medicines or drugs, and they have not received approval from the FDA for the prevention, treatment, or cure of any medical condition, ailment, or disease. We must emphasize that any form of bodily introduction of these products into humans or animals is strictly prohibited by law. It is essential to adhere to these guidelines to ensure compliance with legal and ethical standards in research and experimentation.