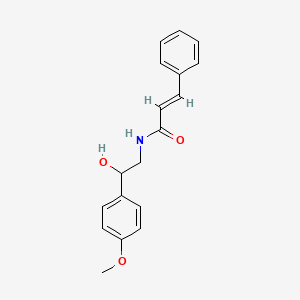
Aegeline
Overview
Description
Aegeline (N-[2-hydroxy-2-(4-methoxyphenyl)ethyl]-3-phenyl-2-propenamide) is a bioactive alkaloid isolated from Aegle marmelos (Bael tree), a plant widely used in Ayurvedic medicine. Structurally, this compound features a phenylpropanoid backbone with methoxy and hydroxyl substitutions, enabling interactions with enzymes and receptors such as monoamine oxidases (MAOs), inducible nitric oxide synthase (iNOS), and β-3 adrenergic receptors . Its pharmacological activities include antihyperlipidemic, antioxidant, anti-inflammatory, and antidepressant effects, as demonstrated in preclinical models of diabetes, colitis, and pain-depression comorbidity .
Preparation Methods
Synthetic Routes and Reaction Conditions: Aegeline can be synthesized through various chemical processes. One common method involves the extraction of Aegle marmelos leaves, followed by isolation and purification using techniques such as ultrahigh-performance liquid chromatography (UHPLC) and nuclear magnetic resonance (NMR) spectroscopy .
Industrial Production Methods: Industrial production of this compound typically involves large-scale extraction from Aegle marmelos leaves. The leaves are harvested, dried, and subjected to solvent extraction. The crude extract is then purified using chromatographic techniques to obtain pure this compound .
Chemical Reactions Analysis
Synthetic Derivatives for β3-Adrenergic Receptor (β3-AR) Agonism
In a study by , novel β3-AR agonists were synthesized by modifying this compound’s structure. The derivative 10C (N-acyl-1-amino-3-arylopropanol) was identified as the most potent, synthesized through:
-
Acylation : Introduction of an acyl group to the amino alcohol backbone.
-
Aromatic Substitution : Incorporation of aryl groups to optimize receptor binding.
Key Reaction Conditions :
-
Reagents : Hexane/ethyl acetate for column chromatography purification.
-
Characterization : NMR (¹H, ¹³C) and high-resolution mass spectrometry (HRMS) confirmed structural integrity.
Derivative | EC₅₀ (β3-AR Agonism) | Key Modifications |
---|---|---|
10C | 447 nM | N-acyl, 3-aryl |
Reactivity of Functional Groups
This compound’s functional groups participate in distinct chemical interactions:
Amide Group Reactivity
-
Hydrolysis : Under acidic/basic conditions, the amide bond can hydrolyze to yield cinnamic acid and 4-methoxyphenylethanolamine.
-
Enzymatic Interactions : this compound inhibits monoamine oxidase-A (MAO-A) via hydrogen bonding (Tyr407) and π-π stacking (Tyr398, Trp119) .
Methoxy Group Reactivity
-
Demethylation : Potential oxidative demethylation under metabolic conditions, though this remains underexplored in current studies.
Spectroscopic Characterization
Post-synthetic modifications and purity assessments rely on advanced spectroscopic techniques:
NMR Data for this compound :
-
¹H NMR (CDCl₃) : δ 7.68 (d, J = 15.6 Hz, 1H, CH=CHCO), 6.81 (d, J = 8.4 Hz, 2H, aromatic), 3.79 (s, 3H, OCH₃).
-
¹³C NMR : δ 167.2 (CONH), 161.1 (C-OCH₃), 144.5 (CH=CHCO).
Mass Spectrometry :
-
HRMS : m/z 312.1702 [M+H]⁺ (calculated for C₁₉H₂₁NO₃: 312.1704).
Molecular Docking and Enzyme Interactions
This compound’s chemical interactions with enzymes highlight its reactive potential:
Target Enzyme | Binding Energy (kcal/mol) | Key Interactions |
---|---|---|
MAO-A | -10.06 | H-bond (Tyr407), π-π stacking |
iNOS | -31.54 | H-bond (Leu264) |
Scientific Research Applications
Antihyperglycemic and Antidyslipidemic Effects
Aegeline has demonstrated significant antihyperglycemic properties, making it a candidate for managing type 2 diabetes. Research indicates that this compound enhances glucose transport via the activation of the Akt signaling pathway, which facilitates GLUT4 translocation in muscle cells. In validated animal models, this compound treatment resulted in a notable decrease in blood glucose levels and improved insulin sensitivity .
Key Findings:
- Mechanism : this compound stimulates glucose uptake through parallel pathways involving Akt and Rac1 signaling .
- Animal Studies : In rats, this compound reduced blood glucose by 12.9% at 5 hours and 16.9% at 24 hours post-administration at a dose of 100 mg/kg .
Weight Management
This compound has been incorporated into dietary supplements aimed at weight loss, such as OxyELITE Pro. Although it has shown potential for fat reduction and metabolic enhancement, its use has been controversial due to reports linking it to liver injury cases .
Considerations:
- Liver Health : While some studies suggest potential hepatotoxicity associated with this compound-containing products, recent evaluations indicate that the evidence does not support a direct causal relationship between this compound and liver damage .
Neuroprotective Properties
Recent studies have highlighted this compound's neuroprotective effects, particularly in models of neurodegenerative diseases such as Parkinson's disease. This compound mimics the yeast SNARE protein Sec22p, which is involved in preventing apoptosis induced by toxic proteins like α-synuclein. This suggests that this compound may have therapeutic implications for synucleinopathies .
Research Insights:
- Cellular Mechanism : this compound inhibits reactive oxygen species (ROS) accumulation and mitochondrial dysfunction in neuronal cells exposed to neurotoxic agents .
Pain-Depression Syndromes
This compound's potential in alleviating pain and depression has been explored through animal models. In experiments involving reserpine-induced pain-depression dyads, this compound significantly improved pain thresholds and reduced immobility behaviors associated with depression .
Experimental Data:
- Dosage : this compound was administered at a dose of 10 mg/kg body weight, showing efficacy in modulating monoamine oxidase-A (MAO-A) activity and inflammatory markers .
Summary Table of this compound Applications
Application Area | Mechanism/Effects | Key Findings/Studies |
---|---|---|
Antihyperglycemic | Enhances glucose transport via Akt signaling | Reduces blood glucose levels significantly |
Weight Management | Potential fat reduction | Controversial due to links with liver injury |
Neuroprotection | Mimics Sec22p to prevent apoptosis | Inhibits ROS and mitochondrial dysfunction |
Pain-Depression | Modulates MAO-A activity | Improves pain thresholds in animal models |
Mechanism of Action
Aegeline exerts its effects through several molecular targets and pathways:
Anti-inflammatory Action: this compound suppresses the NFκB-mediated NLRP3 inflammasome pathway, reducing the expression of pro-inflammatory cytokines such as interleukin-1β and interleukin-18.
Antihyperglycemic Action: this compound enhances glucose transporter type 4 (GLUT4) translocation, increasing glucose uptake in cells.
Antioxidant Action: It reduces oxidative stress by increasing the levels of reduced glutathione and decreasing lipid peroxidation.
Comparison with Similar Compounds
Comparative Analysis with Structurally or Functionally Similar Compounds
Aegeline shares structural and functional similarities with several pharmacologically active compounds. Below is a detailed comparison:
β-3 Adrenergic Receptor Agonists
This compound’s phenylpropanoid structure resembles β-3 adrenergic agonists like Mirabegron, which promote lipolysis and thermogenesis.
Monoamine Oxidase (MAO) Inhibitors
This compound inhibits MAO-A (binding energy: -10.06 kcal/mol) and MAO-B (-10.09 kcal/mol) through hydrogen bonding (Tyr407 in MAO-A, Gln206 in MAO-B) and π-π stacking interactions . Comparatively, Clorgyline (MAO-A inhibitor) and Selegiline (MAO-B inhibitor) exhibit higher selectivity but lack this compound’s dual MAO/iNOS inhibition.
Antihyperlipidemic Agents
This compound reduces serum LDL and triglycerides in Triton-induced hyperlipidemia models, comparable to Fenofibrate (PPAR-α agonist). However, this compound’s dual action (antioxidant + lipid-lowering) distinguishes it from single-target drugs .
Compound | LDL Reduction | Triglyceride Reduction | Antioxidant Activity |
---|---|---|---|
This compound | 35% | 40% | High (↑GSH, ↓lipid peroxidation) |
Fenofibrate | 45% | 50% | Low |
Natural Alkaloids with Anti-inflammatory Effects
This compound suppresses NF-κB-mediated NLRP3 inflammasome activation in colitis models, akin to Berberine. However, this compound’s inhibition of iNOS (binding energy: -31.54 kcal/mol) and IL-6 (↓50% in serum ) exceeds Berberine’s effects in similar models .
Compound | NF-κB Inhibition | iNOS Downregulation | IL-6 Reduction |
---|---|---|---|
This compound | 70% | 60% | 50% |
Berberine | 55% | 40% | 30% |
Biological Activity
Aegeline is a natural compound primarily derived from the leaves and fruits of the plant Aegle marmelos, commonly known as bael. This compound has garnered attention for its diverse biological activities, including potential therapeutic effects against obesity, diabetes, and neurodegenerative diseases. This article synthesizes current research findings, pharmacokinetics, and case studies related to the biological activity of this compound.
Pharmacokinetics and Tissue Distribution
Recent studies have explored the pharmacokinetics of this compound, revealing insights into its absorption and distribution in biological systems. In a study conducted on ND4 mice, this compound was administered at two doses (30 mg/kg and 300 mg/kg). The results indicated:
- Peak Plasma Level : Achieved at of 0.5 hours post-administration.
- Half-Life : Approximately 1.4 hours for the lower dose and 1.3 hours for the higher dose.
- Tissue Concentration : this compound was rapidly eliminated from plasma, with significant concentrations detected in the liver, where it exhibited a half-life of 1.2 hours for the lower dose and 1.7 hours for the higher dose .
Antioxidant Properties
This compound has demonstrated notable antioxidant activity. A study highlighted its ability to mimic the yeast SNARE protein Sec22p, which suppresses apoptosis induced by α-synuclein and Bax proteins—both implicated in neurodegenerative diseases like Parkinson's disease. This protective effect is attributed to this compound's capacity to reduce reactive oxygen species (ROS) levels and preserve mitochondrial function .
Anti-Inflammatory Effects
Research indicates that this compound may exert anti-inflammatory effects by modulating the activity of monoamine oxidase-A (MAO-A) and reducing pro-inflammatory cytokines such as interleukin-6 (IL-6). In vivo studies showed that this compound significantly decreased MAO-A activity and IL-6 levels in reserpine-induced pain-depression models, suggesting its potential as an antidepressant and analgesic agent .
Metabolic Benefits
This compound's role in metabolic regulation has been substantiated by studies demonstrating its effects on insulin sensitivity. A study on synthetic derivatives of this compound found that these compounds acted as β3-adrenergic receptor agonists, promoting lipolysis and improving glucose tolerance in high-fat diet-fed mice. This positions this compound as a candidate for managing insulin resistance and type 2 diabetes .
Case Studies
- Weight Loss Supplements : this compound has been incorporated into various weight loss products, such as OxyELITE Pro. However, its use has raised safety concerns due to reports linking it to acute liver failure in some users .
- Neuroprotection : In yeast models expressing human α-synuclein, this compound effectively prevented apoptosis associated with neurodegenerative processes, highlighting its potential for further exploration in neuroprotective therapies .
Summary of Biological Activities
Q & A
Basic Research Questions
Q. What are the primary pharmacological activities of aegeline in validated animal models, and how are these studies designed?
this compound, an alkaloidal amide isolated from Aegle marmelos leaves, exhibits antihyperglycemic and antidyslipidemic activities in animal models of type 2 diabetes. Studies typically employ high-fat diet (HFD)-fed C57BL6 mice to assess glucose tolerance and insulin sensitivity. Key metrics include fasting blood glucose, lipid profiles, and insulin receptor signaling markers. Experimental designs often include control groups (normal diet), HFD-only groups, and HFD groups treated with this compound or synthetic analogs. Dose-response studies and longitudinal monitoring (e.g., 8-week interventions) are critical for validating efficacy .
Q. What methodologies are used to isolate and characterize this compound from natural sources?
this compound is isolated via solvent extraction (e.g., ethanol or methanol) followed by chromatographic techniques such as column chromatography or HPLC. Structural characterization employs NMR (¹H and ¹³C), mass spectrometry, and IR spectroscopy. Purity validation requires ≥95% purity via HPLC-UV, with comparisons to synthetic standards. For novel compounds, X-ray crystallography may confirm stereochemistry. Known compounds must cross-reference existing literature for identity verification .
Q. Which analytical techniques are standard for quantifying this compound in biological samples?
LC-MS/MS is the gold standard for quantifying this compound in plasma or tissue homogenates due to its sensitivity and specificity. Sample preparation involves protein precipitation (acetonitrile) or solid-phase extraction. Method validation follows ICH guidelines, assessing linearity (1–1000 ng/mL), recovery rates (>80%), and matrix effects. UV-Vis spectroscopy is used for in vitro assays (e.g., enzyme inhibition studies) .
Advanced Research Questions
Q. How can researchers resolve contradictions in reported data on this compound’s molecular targets (e.g., β3AR agonism vs. alternative pathways)?
Discrepancies in mechanistic data require systematic validation:
- Target engagement assays : Use radioligand binding or CRISPR/Cas9 knockout models to confirm β3AR specificity.
- Pathway analysis : Employ phosphoproteomics or transcriptomics to map downstream signaling (e.g., AMPK, PI3K/Akt).
- Cross-species validation : Test human vs. murine cell lines to address species-specific effects.
Contradictions may arise from differences in experimental conditions (e.g., agonist concentration, cell type). Meta-analyses of existing literature should stratify data by methodology .
Q. What experimental design considerations are critical for studying this compound’s effects on insulin resistance in vitro vs. in vivo?
- In vitro : Use insulin-resistant hepatocyte (HepG2) or adipocyte (3T3-L1) models. Pre-treat cells with palmitate or TNF-α to induce insulin resistance. Measure glucose uptake via 2-NBDG assays and IRS-1 phosphorylation via Western blot. Include positive controls (e.g., metformin).
- In vivo : Prioritize translational models like HFD/STZ-induced diabetic rodents. Ensure sample sizes (n ≥ 8/group) to account for biological variability. Monitor body weight, food intake, and glycemic parameters weekly. Terminal assays include hyperinsulinemic-euglycemic clamps for insulin sensitivity .
Q. How can researchers ensure reproducibility when synthesizing this compound analogs for structure-activity relationship (SAR) studies?
- Synthetic protocols : Document reaction conditions (temperature, solvent, catalyst) in detail. Use commercially available reagents with ≥98% purity.
- Characterization : Provide full spectral data (NMR, HRMS) for all intermediates and final compounds.
- Biological assays : Standardize cell lines, passage numbers, and assay buffers across labs. Share raw data via repositories like Zenodo .
Q. What strategies are recommended for integrating multi-omics data to elucidate this compound’s therapeutic mechanisms?
- Transcriptomics : RNA-seq of liver tissue from treated vs. control animals to identify differentially expressed genes (e.g., PPARγ, SREBP1).
- Metabolomics : LC-MS-based profiling of serum/urine to map shifts in lipid/glucose metabolites.
- Network pharmacology : Use tools like STRING or Cytoscape to integrate omics data and predict upstream/downstream targets. Validate hypotheses with siRNA knockdown or inhibitor studies .
Q. How should researchers address ethical and methodological challenges in preclinical toxicity studies of this compound?
- Acute toxicity : Follow OECD Guideline 423, using staggered dosing in rodents (5–2000 mg/kg) with 14-day observation. Monitor organ histopathology (liver, kidneys).
- Ethical compliance : Adhere to ARRIVE 2.0 guidelines for reporting animal studies. Obtain ethics approval and minimize animal numbers via power analysis.
- Data transparency : Publish negative results (e.g., lack of efficacy at certain doses) to avoid publication bias .
Q. What frameworks (e.g., FINER, PICO) are applicable for formulating hypothesis-driven research questions on this compound?
- FINER Criteria : Ensure questions are Feasible (e.g., access to Aegle marmelos extracts), Interesting (novel mechanisms), Novel (unexplored signaling pathways), Ethical (animal welfare compliance), and Relevant (diabetes therapeutics).
- PICO Framework : Define Population (e.g., HFD mice), Intervention (this compound dosage), Comparison (metformin), and Outcome (HbA1c reduction). Use these to refine questions and align with grant funding priorities .
Q. How can systematic reviews address gaps in the literature on this compound’s therapeutic potential?
- Search strategy : Use PRISMA guidelines to query PubMed, Scopus, and Embase with terms like “this compound AND (diabetes OR insulin resistance).” Include preclinical studies and exclude patents/industrial reports.
- Risk of bias : Assess studies via SYRCLE’s tool for animal research. Highlight limitations (e.g., small sample sizes, lack of blinding).
- Meta-analysis : Pool data on glucose tolerance using random-effects models. Report heterogeneity via I² statistics .
Properties
IUPAC Name |
(E)-N-[2-hydroxy-2-(4-methoxyphenyl)ethyl]-3-phenylprop-2-enamide | |
---|---|---|
Source | PubChem | |
URL | https://pubchem.ncbi.nlm.nih.gov | |
Description | Data deposited in or computed by PubChem | |
InChI |
InChI=1S/C18H19NO3/c1-22-16-10-8-15(9-11-16)17(20)13-19-18(21)12-7-14-5-3-2-4-6-14/h2-12,17,20H,13H2,1H3,(H,19,21)/b12-7+ | |
Source | PubChem | |
URL | https://pubchem.ncbi.nlm.nih.gov | |
Description | Data deposited in or computed by PubChem | |
InChI Key |
QRFDENJATPJOKG-KPKJPENVSA-N | |
Source | PubChem | |
URL | https://pubchem.ncbi.nlm.nih.gov | |
Description | Data deposited in or computed by PubChem | |
Canonical SMILES |
COC1=CC=C(C=C1)C(CNC(=O)C=CC2=CC=CC=C2)O | |
Source | PubChem | |
URL | https://pubchem.ncbi.nlm.nih.gov | |
Description | Data deposited in or computed by PubChem | |
Isomeric SMILES |
COC1=CC=C(C=C1)C(CNC(=O)/C=C/C2=CC=CC=C2)O | |
Source | PubChem | |
URL | https://pubchem.ncbi.nlm.nih.gov | |
Description | Data deposited in or computed by PubChem | |
Molecular Formula |
C18H19NO3 | |
Source | PubChem | |
URL | https://pubchem.ncbi.nlm.nih.gov | |
Description | Data deposited in or computed by PubChem | |
DSSTOX Substance ID |
DTXSID101318019 | |
Record name | (±)-Aegeline | |
Source | EPA DSSTox | |
URL | https://comptox.epa.gov/dashboard/DTXSID101318019 | |
Description | DSSTox provides a high quality public chemistry resource for supporting improved predictive toxicology. | |
Molecular Weight |
297.3 g/mol | |
Source | PubChem | |
URL | https://pubchem.ncbi.nlm.nih.gov | |
Description | Data deposited in or computed by PubChem | |
Physical Description |
Solid | |
Record name | (±)-Aegeline | |
Source | Human Metabolome Database (HMDB) | |
URL | http://www.hmdb.ca/metabolites/HMDB0033435 | |
Description | The Human Metabolome Database (HMDB) is a freely available electronic database containing detailed information about small molecule metabolites found in the human body. | |
Explanation | HMDB is offered to the public as a freely available resource. Use and re-distribution of the data, in whole or in part, for commercial purposes requires explicit permission of the authors and explicit acknowledgment of the source material (HMDB) and the original publication (see the HMDB citing page). We ask that users who download significant portions of the database cite the HMDB paper in any resulting publications. | |
CAS No. |
456-12-2, 37791-13-2 | |
Record name | (±)-Aegeline | |
Source | CAS Common Chemistry | |
URL | https://commonchemistry.cas.org/detail?cas_rn=456-12-2 | |
Description | CAS Common Chemistry is an open community resource for accessing chemical information. Nearly 500,000 chemical substances from CAS REGISTRY cover areas of community interest, including common and frequently regulated chemicals, and those relevant to high school and undergraduate chemistry classes. This chemical information, curated by our expert scientists, is provided in alignment with our mission as a division of the American Chemical Society. | |
Explanation | The data from CAS Common Chemistry is provided under a CC-BY-NC 4.0 license, unless otherwise stated. | |
Record name | Aegeline | |
Source | ChemIDplus | |
URL | https://pubchem.ncbi.nlm.nih.gov/substance/?source=chemidplus&sourceid=0000456122 | |
Description | ChemIDplus is a free, web search system that provides access to the structure and nomenclature authority files used for the identification of chemical substances cited in National Library of Medicine (NLM) databases, including the TOXNET system. | |
Record name | (±)-Aegeline | |
Source | EPA DSSTox | |
URL | https://comptox.epa.gov/dashboard/DTXSID101318019 | |
Description | DSSTox provides a high quality public chemistry resource for supporting improved predictive toxicology. | |
Record name | AEGELINE | |
Source | FDA Global Substance Registration System (GSRS) | |
URL | https://gsrs.ncats.nih.gov/ginas/app/beta/substances/60T59LN3SG | |
Description | The FDA Global Substance Registration System (GSRS) enables the efficient and accurate exchange of information on what substances are in regulated products. Instead of relying on names, which vary across regulatory domains, countries, and regions, the GSRS knowledge base makes it possible for substances to be defined by standardized, scientific descriptions. | |
Explanation | Unless otherwise noted, the contents of the FDA website (www.fda.gov), both text and graphics, are not copyrighted. They are in the public domain and may be republished, reprinted and otherwise used freely by anyone without the need to obtain permission from FDA. Credit to the U.S. Food and Drug Administration as the source is appreciated but not required. | |
Record name | (±)-Aegeline | |
Source | Human Metabolome Database (HMDB) | |
URL | http://www.hmdb.ca/metabolites/HMDB0033435 | |
Description | The Human Metabolome Database (HMDB) is a freely available electronic database containing detailed information about small molecule metabolites found in the human body. | |
Explanation | HMDB is offered to the public as a freely available resource. Use and re-distribution of the data, in whole or in part, for commercial purposes requires explicit permission of the authors and explicit acknowledgment of the source material (HMDB) and the original publication (see the HMDB citing page). We ask that users who download significant portions of the database cite the HMDB paper in any resulting publications. | |
Melting Point |
176 °C | |
Record name | (±)-Aegeline | |
Source | Human Metabolome Database (HMDB) | |
URL | http://www.hmdb.ca/metabolites/HMDB0033435 | |
Description | The Human Metabolome Database (HMDB) is a freely available electronic database containing detailed information about small molecule metabolites found in the human body. | |
Explanation | HMDB is offered to the public as a freely available resource. Use and re-distribution of the data, in whole or in part, for commercial purposes requires explicit permission of the authors and explicit acknowledgment of the source material (HMDB) and the original publication (see the HMDB citing page). We ask that users who download significant portions of the database cite the HMDB paper in any resulting publications. | |
Retrosynthesis Analysis
AI-Powered Synthesis Planning: Our tool employs the Template_relevance Pistachio, Template_relevance Bkms_metabolic, Template_relevance Pistachio_ringbreaker, Template_relevance Reaxys, Template_relevance Reaxys_biocatalysis model, leveraging a vast database of chemical reactions to predict feasible synthetic routes.
One-Step Synthesis Focus: Specifically designed for one-step synthesis, it provides concise and direct routes for your target compounds, streamlining the synthesis process.
Accurate Predictions: Utilizing the extensive PISTACHIO, BKMS_METABOLIC, PISTACHIO_RINGBREAKER, REAXYS, REAXYS_BIOCATALYSIS database, our tool offers high-accuracy predictions, reflecting the latest in chemical research and data.
Strategy Settings
Precursor scoring | Relevance Heuristic |
---|---|
Min. plausibility | 0.01 |
Model | Template_relevance |
Template Set | Pistachio/Bkms_metabolic/Pistachio_ringbreaker/Reaxys/Reaxys_biocatalysis |
Top-N result to add to graph | 6 |
Feasible Synthetic Routes
Disclaimer and Information on In-Vitro Research Products
Please be aware that all articles and product information presented on BenchChem are intended solely for informational purposes. The products available for purchase on BenchChem are specifically designed for in-vitro studies, which are conducted outside of living organisms. In-vitro studies, derived from the Latin term "in glass," involve experiments performed in controlled laboratory settings using cells or tissues. It is important to note that these products are not categorized as medicines or drugs, and they have not received approval from the FDA for the prevention, treatment, or cure of any medical condition, ailment, or disease. We must emphasize that any form of bodily introduction of these products into humans or animals is strictly prohibited by law. It is essential to adhere to these guidelines to ensure compliance with legal and ethical standards in research and experimentation.