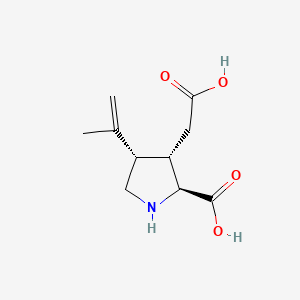
Kainic acid
- Click on QUICK INQUIRY to receive a quote from our team of experts.
- With the quality product at a COMPETITIVE price, you can focus more on your research.
Overview
Description
Kainic acid is a naturally occurring organic acid found in certain species of red seaweed, particularly in the genus Digenea. It was first isolated in 1953 by Japanese scientists from the seaweed Digenea simplex. This compound is known for its potent neuroexcitatory properties, acting as an agonist for kainate receptors, a subtype of ionotropic glutamate receptors in the central nervous system .
Preparation Methods
Synthetic Routes and Reaction Conditions: Kainic acid can be synthesized through various methods. One common synthetic route involves the use of a tritiated Wittig reagent. The synthesis typically includes steps such as the protection of functional groups, formation of key intermediates, and final deprotection to yield this compound. For example, the synthesis may involve the following steps:
- Protection of the amino group using Boc-ON in a dioxane/water mixture at room temperature.
- Formation of a key intermediate through reactions with methanol and ozone.
- Final deprotection using trifluoroacetic acid followed by neutralization with sodium hydroxide .
Industrial Production Methods: Industrial production of this compound often involves extraction from natural sources, such as the red seaweed Digenea simplex. The extraction process includes drying the seaweed, followed by solvent extraction and purification steps to isolate this compound in its pure form .
Chemical Reactions Analysis
Types of Reactions: Kainic acid undergoes various chemical reactions, including:
Oxidation: this compound can be oxidized to form different derivatives.
Reduction: Reduction reactions can modify the functional groups of this compound.
Substitution: Substitution reactions can introduce new functional groups into the this compound molecule.
Common Reagents and Conditions:
Oxidation: Common oxidizing agents include ozone and other peroxides.
Reduction: Reducing agents such as sodium borohydride can be used.
Substitution: Substitution reactions often involve nucleophiles like organolithium reagents.
Major Products: The major products formed from these reactions include various derivatives of this compound, which can be used for further research and applications .
Scientific Research Applications
Kainic Acid in Epilepsy Research
This compound is widely used to induce seizures in animal models, particularly for studying temporal lobe epilepsy (TLE). The administration of KA results in behavioral seizures and neuropathological changes that mirror those seen in human epilepsy.
Case Study: Temporal Lobe Epilepsy Model
- Methodology : KA can be administered via systemic or intracerebral routes, with different protocols yielding varying results in seizure severity and brain damage.
- Findings : Research has demonstrated that KA-induced seizures lead to significant neuronal loss in the hippocampus, particularly affecting the CA3 region . This model has been instrumental in understanding the mechanisms underlying epileptogenesis and ictogenesis.
Neurodegenerative Disease Models
This compound is also utilized to study various neurodegenerative diseases, including Huntington's disease (HD), Alzheimer's disease (AD), and amyotrophic lateral sclerosis (ALS). Its excitotoxic properties allow researchers to investigate the pathways involved in neuronal death and degeneration.
Case Study: Huntington's Disease
- Methodology : Stereotaxic microinjections of KA into specific brain regions create selective lesions that mimic the pathological features of HD.
- Findings : Studies have shown that KA lesions result in motor deficits and cognitive impairments similar to those observed in HD patients . This application has led to insights into the excitotoxic mechanisms contributing to neurodegeneration.
Spinal Cord Injury Research
This compound is increasingly being used as a model for spinal cord injury (SCI) due to its cost-effectiveness and reproducibility compared to traditional methods.
Case Study: Inducing Spinal Cord Injury
- Methodology : Intra-cisternal or intra-spinal administration of KA induces paraplegia and locomotor deficits in rats.
- Findings : Research indicates that KA-induced SCI models exhibit similar clinical manifestations as seen in human conditions, providing a valuable tool for testing therapeutic interventions . The ability to adjust the concentration and administration rate allows researchers to control the severity of injury.
Mechanistic Studies of Excitotoxicity
This compound serves as a critical tool for exploring excitotoxicity—the process by which neurons are damaged or killed by excessive stimulation by neurotransmitters such as glutamate.
Mechanisms Investigated
- Neuronal Cell Death : KA induces robust depolarization and subsequent cell death, facilitating studies on excitatory neurotransmission pathways .
- Neuroprotective Strategies : Researchers are investigating potential neuroprotective agents against KA-induced damage, contributing to the development of therapies for neurodegenerative diseases .
Mechanism of Action
Kainic acid exerts its effects by binding to kainate receptors, a subtype of ionotropic glutamate receptors. These receptors are ligand-gated ion channels that, when activated by this compound, allow the influx of sodium ions into the neuron, leading to depolarization and excitatory postsynaptic potentials. In high concentrations, this compound can cause excitotoxicity, leading to neuronal death .
Comparison with Similar Compounds
Kainic acid is structurally similar to other excitatory amino acids, such as glutamic acid and domoic acid. it is unique in its high affinity for kainate receptors. Similar compounds include:
Glutamic Acid: A major excitatory neurotransmitter in the central nervous system.
Domoic Acid: Another marine-derived excitatory amino acid that binds to kainate receptors but has a different toxicity profile.
Dysiherbaine: A sponge-derived excitatory amino acid that also targets kainate receptors.
This compound’s specificity for kainate receptors and its potent neuroexcitatory properties make it a valuable tool in neuroscience research, distinguishing it from other similar compounds .
Biological Activity
Kainic acid (KA) is a potent neurotoxin and a selective agonist of kainate-type ionotropic glutamate receptors. It has been extensively studied for its role in neurodegenerative diseases, excitotoxicity, and as a model for epilepsy. This article provides a comprehensive overview of the biological activity of this compound, including its mechanisms of action, effects on neuronal cells, and implications for research.
This compound primarily acts by activating kainate receptors, which are a subtype of glutamate receptors. The binding of KA leads to the following biological activities:
- Neuronal Excitation : KA induces excitotoxicity by increasing intracellular calcium levels, resulting in apoptosis and necrosis of neurons. This mechanism is particularly evident in hippocampal regions (CA1, CA3) and the dentate gyrus .
- Oxidative Stress : KA administration increases the production of reactive oxygen species (ROS), leading to mitochondrial dysfunction and lipid peroxidation . This oxidative stress is implicated in various neurodegenerative conditions.
- Inflammatory Response : Systemic injection of KA activates glial cells, triggering inflammatory responses that are characteristic of neurodegenerative diseases .
Experimental Models and Findings
This compound is widely used in animal models to study epilepsy and neurodegeneration. Below is a summary of key findings from various studies:
Case Studies
-
This compound-Induced Seizures in Mice :
A comparative study involving three strains of DBA/2 mice demonstrated consistent seizure responses to KA injections. The study evaluated survival rates and seizure phenotypes, confirming that all strains exhibited similar trends in behavior following KA administration . -
Neuroprotective Effects of Melatonin :
Research indicated that melatonin could protect hippocampal neurons from KA-induced damage. This suggests potential therapeutic avenues for mitigating excitotoxic effects associated with KA exposure . -
Lipid Peroxidation Studies :
Investigations into lipid peroxidation highlighted the role of oxidative stress as a mediator of neuronal damage in response to KA. The findings underscore the importance of antioxidants in potentially counteracting these effects .
Implications for Research
The biological activity of this compound serves as a critical model for understanding excitotoxicity and neurodegeneration. Its ability to mimic pathological processes observed in human diseases such as Alzheimer's and epilepsy makes it invaluable for research into therapeutic interventions.
- Neurodegenerative Disease Models : KA-induced excitotoxicity models are instrumental in studying the underlying mechanisms of diseases like Alzheimer's, where glutamate signaling plays a pivotal role.
- Therapeutic Development : Understanding KA's mechanisms can guide the development of neuroprotective strategies aimed at mitigating excitotoxic damage.
Properties
CAS No. |
59905-23-6 |
---|---|
Molecular Formula |
C10H15NO4 |
Molecular Weight |
213.23 g/mol |
IUPAC Name |
(2R,3S,4S)-3-(carboxymethyl)-4-prop-1-en-2-ylpyrrolidine-2-carboxylic acid |
InChI |
InChI=1S/C10H15NO4/c1-5(2)7-4-11-9(10(14)15)6(7)3-8(12)13/h6-7,9,11H,1,3-4H2,2H3,(H,12,13)(H,14,15)/t6-,7+,9+/m0/s1 |
InChI Key |
VLSMHEGGTFMBBZ-LKEWCRSYSA-N |
SMILES |
CC(=C)C1CNC(C1CC(=O)O)C(=O)O |
Isomeric SMILES |
CC(=C)[C@H]1CN[C@H]([C@H]1CC(=O)O)C(=O)O |
Canonical SMILES |
CC(=C)C1CNC(C1CC(=O)O)C(=O)O |
Origin of Product |
United States |
Retrosynthesis Analysis
AI-Powered Synthesis Planning: Our tool employs the Template_relevance Pistachio, Template_relevance Bkms_metabolic, Template_relevance Pistachio_ringbreaker, Template_relevance Reaxys, Template_relevance Reaxys_biocatalysis model, leveraging a vast database of chemical reactions to predict feasible synthetic routes.
One-Step Synthesis Focus: Specifically designed for one-step synthesis, it provides concise and direct routes for your target compounds, streamlining the synthesis process.
Accurate Predictions: Utilizing the extensive PISTACHIO, BKMS_METABOLIC, PISTACHIO_RINGBREAKER, REAXYS, REAXYS_BIOCATALYSIS database, our tool offers high-accuracy predictions, reflecting the latest in chemical research and data.
Strategy Settings
Precursor scoring | Relevance Heuristic |
---|---|
Min. plausibility | 0.01 |
Model | Template_relevance |
Template Set | Pistachio/Bkms_metabolic/Pistachio_ringbreaker/Reaxys/Reaxys_biocatalysis |
Top-N result to add to graph | 6 |
Feasible Synthetic Routes
Disclaimer and Information on In-Vitro Research Products
Please be aware that all articles and product information presented on BenchChem are intended solely for informational purposes. The products available for purchase on BenchChem are specifically designed for in-vitro studies, which are conducted outside of living organisms. In-vitro studies, derived from the Latin term "in glass," involve experiments performed in controlled laboratory settings using cells or tissues. It is important to note that these products are not categorized as medicines or drugs, and they have not received approval from the FDA for the prevention, treatment, or cure of any medical condition, ailment, or disease. We must emphasize that any form of bodily introduction of these products into humans or animals is strictly prohibited by law. It is essential to adhere to these guidelines to ensure compliance with legal and ethical standards in research and experimentation.