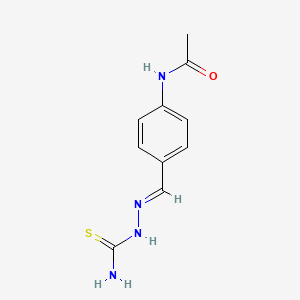
Thiacetazone
Overview
Description
Mechanism of Action
Thiacetazone, also known as Thioacetazone or AMITHIOZONE, is an oral antibiotic that was formerly used in the treatment of tuberculosis . This article will delve into the mechanism of action, biochemical pathways, pharmacokinetics, and the result of action of this compound.
Target of Action
The biological target of this compound has proven elusive . It is thought to interfere with mycolic acid synthesis . Mycolic acids are key components of the highly hydrophobic mycobacterial cell wall .
Mode of Action
This compound is a prodrug that requires activation by a bacterially encoded monoxygenase, EthA, to be active . EthA also activates ethionamide, and it would be expected that mutations in the corresponding gene, etha, would result in complete cross-resistance .
Biochemical Pathways
This compound and its chemical analogues inhibit mycolic acid cyclopropanation . Cyclopropanated mycolic acids are key factors participating in cell envelope permeability, host immunomodulation, and persistence of M. tuberculosis . Overexpression of cmaA2, mmaA2, or pcaA in mycobacteria partially reversed the effects of this compound and its analogue on mycolic acid cyclopropanation, suggesting that the drugs act directly on cyclopropane mycolic acid synthases (CMASs) .
Pharmacokinetics
It is known that this compound was used orally in the treatment of tuberculosis .
Result of Action
The result of this compound’s action is a significant loss of cyclopropanation in both the α- and oxygenated mycolate sub-types . This leads to dramatic changes in the content and ratio of mycolic acids in the mycobacteria .
Action Environment
The action of this compound can be influenced by environmental factors such as the presence of other drugs. For instance, the use of this compound is declining because it can cause severe (sometimes fatal) skin reactions in HIV positive patients .
Biochemical Analysis
Biochemical Properties
Thiacetazone plays a significant role in biochemical reactions, particularly in the metabolism of mycolic acids . Mycolic acids are a complex mixture of branched, long-chain fatty acids, which are key components of the highly hydrophobic mycobacterial cell wall . This compound inhibits the cyclopropanation of cell wall mycolic acids in mycobacteria . This process involves the action of cyclopropane mycolic acid synthases (CMASs), a family of S-adenosyl-methionine-dependent methyl transferases .
Cellular Effects
This compound exerts its effects on various types of cells and cellular processes. It influences cell function by inhibiting the synthesis of mycolic acids, which are key factors participating in cell envelope permeability, host immunomodulation, and persistence of M. tuberculosis .
Molecular Mechanism
This compound exerts its effects at the molecular level by inhibiting the action of CMASs . These enzymes modify double bonds at specific sites on mycolic acid precursors . Overexpression of cmaA2, mmaA2, or pcaA in mycobacteria partially reverses the effects of this compound on mycolic acid cyclopropanation, suggesting that this compound acts directly on CMASs .
Temporal Effects in Laboratory Settings
The effects of this compound on mycolic acid cyclopropanation change over time in laboratory settings . Dramatic changes in the content and ratio of mycolic acids were observed after treatment with this compound .
Dosage Effects in Animal Models
The effects of this compound on animal models vary with different dosages
Metabolic Pathways
This compound is involved in the metabolic pathway of mycolic acid synthesis . It interacts with CMASs, which are involved in the modification of double bonds at specific sites on mycolic acid precursors .
Preparation Methods
Thiacetazone can be synthesized through the reaction of thiosemicarbazide with 4-acetylaminobenzaldehyde . The reaction typically involves dissolving thiosemicarbazide in ethanol and adding 4-acetylaminobenzaldehyde under acidic conditions. The mixture is then heated to facilitate the reaction, resulting in the formation of thioacetazone . Industrial production methods are similar but often involve larger-scale equipment and more stringent controls to ensure purity and yield.
Chemical Reactions Analysis
Thiacetazone undergoes several types of chemical reactions, including:
Oxidation: this compound can be oxidized to form various sulfoxides and sulfones.
Reduction: It can be reduced to form thiosemicarbazide derivatives.
Substitution: this compound can undergo nucleophilic substitution reactions, particularly at the thioamide group.
Common reagents used in these reactions include oxidizing agents like hydrogen peroxide and reducing agents like sodium borohydride . The major products formed from these reactions depend on the specific conditions and reagents used.
Scientific Research Applications
Thiacetazone has been studied extensively for its applications in various fields:
Comparison with Similar Compounds
Thiacetazone is similar to other thiourea-containing compounds such as thiocarlide and enzalutamide . its unique mechanism of action and low cost make it distinct. Unlike ethambutol, which is also used to prevent resistance in tuberculosis treatment, thioacetazone is more prone to causing severe skin reactions, particularly in HIV-positive patients .
Similar Compounds
Thiocarlide: Another thiourea-containing antitubercular agent.
Enzalutamide: A thiourea derivative used in the treatment of prostate cancer.
This compound’s unique properties and historical significance continue to make it a subject of interest in scientific research and industrial applications.
Properties
CAS No. |
910379-02-1 |
---|---|
Molecular Formula |
C10H12N4OS |
Molecular Weight |
236.30 g/mol |
IUPAC Name |
N-[4-[(Z)-(carbamothioylhydrazinylidene)methyl]phenyl]acetamide |
InChI |
InChI=1S/C10H12N4OS/c1-7(15)13-9-4-2-8(3-5-9)6-12-14-10(11)16/h2-6H,1H3,(H,13,15)(H3,11,14,16)/b12-6- |
InChI Key |
SRVJKTDHMYAMHA-SDQBBNPISA-N |
SMILES |
CC(=O)NC1=CC=C(C=C1)C=NNC(=S)N |
Isomeric SMILES |
CC(=O)NC1=CC=C(C=C1)/C=N\NC(=S)N |
Canonical SMILES |
CC(=O)NC1=CC=C(C=C1)C=NNC(=S)N |
Origin of Product |
United States |
Retrosynthesis Analysis
AI-Powered Synthesis Planning: Our tool employs the Template_relevance Pistachio, Template_relevance Bkms_metabolic, Template_relevance Pistachio_ringbreaker, Template_relevance Reaxys, Template_relevance Reaxys_biocatalysis model, leveraging a vast database of chemical reactions to predict feasible synthetic routes.
One-Step Synthesis Focus: Specifically designed for one-step synthesis, it provides concise and direct routes for your target compounds, streamlining the synthesis process.
Accurate Predictions: Utilizing the extensive PISTACHIO, BKMS_METABOLIC, PISTACHIO_RINGBREAKER, REAXYS, REAXYS_BIOCATALYSIS database, our tool offers high-accuracy predictions, reflecting the latest in chemical research and data.
Strategy Settings
Precursor scoring | Relevance Heuristic |
---|---|
Min. plausibility | 0.01 |
Model | Template_relevance |
Template Set | Pistachio/Bkms_metabolic/Pistachio_ringbreaker/Reaxys/Reaxys_biocatalysis |
Top-N result to add to graph | 6 |
Feasible Synthetic Routes
Disclaimer and Information on In-Vitro Research Products
Please be aware that all articles and product information presented on BenchChem are intended solely for informational purposes. The products available for purchase on BenchChem are specifically designed for in-vitro studies, which are conducted outside of living organisms. In-vitro studies, derived from the Latin term "in glass," involve experiments performed in controlled laboratory settings using cells or tissues. It is important to note that these products are not categorized as medicines or drugs, and they have not received approval from the FDA for the prevention, treatment, or cure of any medical condition, ailment, or disease. We must emphasize that any form of bodily introduction of these products into humans or animals is strictly prohibited by law. It is essential to adhere to these guidelines to ensure compliance with legal and ethical standards in research and experimentation.