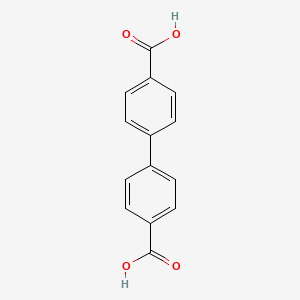
Biphenyl-4,4'-dicarboxylic acid
Overview
Description
Biphenyl-4,4’-dicarboxylic acid is an aromatic dicarboxylic acid with the molecular formula C14H10O4. It is a white to light beige powder that belongs to the class of monomers known as aromatic dicarboxylic acids. This compound is used to prepare polymers that exhibit excellent thermal stability, making them suitable for high-temperature applications. Additionally, these polymers demonstrate high electrical insulation properties due to their low dielectric constant and high breakdown strength .
Mechanism of Action
Target of Action
Biphenyl-4,4’-dicarboxylic acid (BPDC) is primarily used as a monomer in the synthesis of BPDC-based polymers . These polymers exhibit excellent thermal stability, making them suitable for applications where high-temperature resistance is required . The primary target of BPDC is therefore the polymer matrix, where it contributes to the overall stability and performance of the polymer.
Mode of Action
The mode of action of BPDC involves its incorporation into the polymer matrix. When added into a solution, BPDC molecules can ionize and construct a monolayer liquid membrane . In the presence of metal ions, such as nickel, the ions can coordinate with the carboxyl ions on the surface of the BPDC membrane, forming an interconnected nanoplate morphology . This interaction enhances the thermal stability and electrical insulation properties of the resulting polymer .
Biochemical Pathways
Its primary role is in the formation of polymers with enhanced thermal stability and electrical insulation properties .
Result of Action
The result of BPDC’s action is the formation of polymers with enhanced thermal stability and electrical insulation properties . These polymers can be used in various applications, including in the electrical and electronics industry for insulation, encapsulation, and fabrication of high-performance electronic devices .
Action Environment
The action of BPDC is influenced by environmental factors such as temperature, pH, and the presence of metal ions. For example, the formation of the BPDC membrane and its interaction with metal ions can be influenced by the pH of the solution . Additionally, the thermal stability of the resulting polymer is a key property of BPDC, suggesting that it can function effectively even in high-temperature environments .
Biochemical Analysis
Biochemical Properties
Biphenyl-4,4’-dicarboxylic acid plays a significant role in biochemical reactions, particularly in the synthesis of polymers and metal-organic frameworks. It interacts with enzymes such as esterases and carboxylases, which facilitate the formation of ester and carboxylate groups, respectively. These interactions are crucial for the polymerization process and the formation of stable metal-organic frameworks . Additionally, biphenyl-4,4’-dicarboxylic acid can interact with proteins and other biomolecules, influencing their structural stability and function.
Cellular Effects
Biphenyl-4,4’-dicarboxylic acid affects various types of cells and cellular processes. It has been shown to influence cell signaling pathways, gene expression, and cellular metabolism. For instance, biphenyl-4,4’-dicarboxylic acid can modulate the activity of transcription factors, leading to changes in gene expression. It also affects cellular metabolism by altering the activity of metabolic enzymes, which can impact the overall metabolic flux within the cell .
Molecular Mechanism
The molecular mechanism of biphenyl-4,4’-dicarboxylic acid involves its binding interactions with biomolecules, enzyme inhibition or activation, and changes in gene expression. Biphenyl-4,4’-dicarboxylic acid can bind to specific enzymes, either inhibiting or activating their activity. This binding can lead to changes in the enzyme’s conformation and function, ultimately affecting the biochemical pathways in which the enzyme is involved. Additionally, biphenyl-4,4’-dicarboxylic acid can influence gene expression by interacting with transcription factors and other regulatory proteins .
Temporal Effects in Laboratory Settings
In laboratory settings, the effects of biphenyl-4,4’-dicarboxylic acid can change over time. The compound’s stability and degradation are important factors to consider. Biphenyl-4,4’-dicarboxylic acid is relatively stable under standard laboratory conditions, but it can degrade over time, leading to changes in its biochemical activity. Long-term studies have shown that biphenyl-4,4’-dicarboxylic acid can have lasting effects on cellular function, including alterations in gene expression and metabolic activity .
Dosage Effects in Animal Models
The effects of biphenyl-4,4’-dicarboxylic acid vary with different dosages in animal models. At low doses, the compound can have beneficial effects, such as enhancing metabolic activity and improving cellular function. At high doses, biphenyl-4,4’-dicarboxylic acid can exhibit toxic or adverse effects, including cellular damage and disruption of normal metabolic processes. Threshold effects have been observed, where the compound’s impact on cellular function changes significantly at certain dosage levels .
Metabolic Pathways
Biphenyl-4,4’-dicarboxylic acid is involved in various metabolic pathways, interacting with enzymes and cofactors that facilitate its conversion into different metabolites. The compound can affect metabolic flux by altering the activity of key metabolic enzymes, leading to changes in the levels of specific metabolites. These interactions are crucial for maintaining cellular homeostasis and ensuring the proper functioning of metabolic pathways .
Transport and Distribution
Within cells and tissues, biphenyl-4,4’-dicarboxylic acid is transported and distributed through specific transporters and binding proteins. These interactions influence the compound’s localization and accumulation within different cellular compartments. The transport and distribution of biphenyl-4,4’-dicarboxylic acid are essential for its biochemical activity and its ability to exert its effects on cellular function .
Subcellular Localization
The subcellular localization of biphenyl-4,4’-dicarboxylic acid is determined by targeting signals and post-translational modifications that direct it to specific compartments or organelles. This localization is critical for the compound’s activity and function, as it allows biphenyl-4,4’-dicarboxylic acid to interact with specific biomolecules and participate in localized biochemical reactions. The compound’s subcellular distribution can also affect its overall impact on cellular function and metabolism .
Preparation Methods
Biphenyl-4,4’-dicarboxylic acid can be synthesized through various methods. One common synthetic route involves the isolation of a mixture of diisopropylbiphenyls from a reaction mixture obtained by reacting biphenyl with propylene. The 4,4’-diisopropylbiphenyl is then crystallized from the mixture and oxidized with molecular oxygen in the presence of an oxidation catalyst, such as a cobalt or manganese catalyst, in a solvent containing at least 50% of an aliphatic monocarboxylic acid . Another method involves the hydrothermal synthesis of nickel-based metal-organic frameworks using biphenyl-4,4’-dicarboxylic acid as an organic linker .
Chemical Reactions Analysis
Biphenyl-4,4’-dicarboxylic acid undergoes various chemical reactions, including oxidation, reduction, and substitution. Common reagents and conditions used in these reactions include molecular oxygen, cobalt or manganese catalysts, and aliphatic monocarboxylic acids. The major products formed from these reactions include 4,4’-diisopropylbiphenyl and its oxidation intermediates . Additionally, biphenyl-4,4’-dicarboxylic acid can be used in the synthesis of metal-organic frameworks through post-synthetic linker exchange and orthogonal click chemistry .
Scientific Research Applications
Biphenyl-4,4’-dicarboxylic acid has a wide range of scientific research applications. In chemistry, it is used as a key component in the synthesis of nematic aromatic polyesters, which are valuable in the development of liquid crystal polymers for electronics, optical devices, automotive, and textiles . In biology and medicine, it serves as a building block in the synthesis of various pharmaceutical compounds and materials with biological activity. It is also utilized in the development of drug delivery systems and biomaterials due to its chemical properties . In industry, biphenyl-4,4’-dicarboxylic acid is used in the preparation of high-performance polymers and metal-organic frameworks for applications such as gas storage, catalysis, sensing, and separation technology .
Comparison with Similar Compounds
Biphenyl-4,4’-dicarboxylic acid can be compared with other similar compounds, such as terephthalic acid, isophthalic acid, and 2,6-naphthalenedicarboxylic acid. These compounds also belong to the class of aromatic dicarboxylic acids and are used in the synthesis of high-performance polymers. biphenyl-4,4’-dicarboxylic acid is unique due to its ability to form stable metal-organic frameworks with tunable pore sizes, making it suitable for a wide range of applications in gas storage, catalysis, and sensing .
Similar Compounds::- Terephthalic acid
- Isophthalic acid
- 2,6-Naphthalenedicarboxylic acid
- Biphenyl-4-carboxylic acid
- Dimethyl biphenyl-4,4’-dicarboxylate
- 4,4’-Stilbenedicarboxylic acid
Biological Activity
Biphenyl-4,4'-dicarboxylic acid (BPDC), also known as 4,4'-bibenzoic acid, is a compound of significant interest in various fields, including materials science and biochemistry. This article explores the biological activity of BPDC, focusing on its biochemical properties, mechanisms of action, and applications in biomedical research.
- Molecular Formula : CHO
- Molecular Weight : 242.23 g/mol
- Melting Point : >300 °C
- Boiling Point : 482.5 °C
- Density : 1.3 g/cm³
BPDC consists of two carboxylic acid groups attached to a biphenyl structure, which contributes to its unique chemical reactivity and potential biological applications .
BPDC primarily acts as a monomer in the synthesis of polymers and other complex materials. Its biological activity can be attributed to several mechanisms:
- Polymerization : BPDC can form covalent bonds during polymerization processes, leading to the development of high-performance polymers with enhanced thermal stability and electrical insulation properties.
- Interaction with Biological Molecules : BPDC can interact with various biomolecules, forming complexes that may influence biochemical pathways. For instance, it has been shown to participate in the formation of metal-organic frameworks (MOFs), which can be utilized in drug delivery systems and catalysis .
- Antimicrobial Activity : Research has indicated that derivatives of biphenyl-4-carboxylic acid exhibit antimicrobial properties against specific Gram-negative bacteria such as Escherichia coli and Pseudomonas aeruginosa. This suggests that BPDC and its derivatives could be explored for potential therapeutic applications .
Table 1: Summary of Biological Activities of BPDC Derivatives
Compound Type | Activity | Target Organisms/Applications |
---|---|---|
Biphenyl-4-carboxylic acid | Antimicrobial | E. coli, P. aeruginosa |
BPDC-based polymers | Thermal stability | Electronic devices, high-temperature applications |
Metal-organic frameworks (MOFs) | Drug delivery | Various pharmaceutical applications |
Case Studies
- Antimicrobial Studies : A study published in 2010 evaluated a series of biphenyl-4-carboxylic acid hydrazide-hydrazones for their antimicrobial activity. The results indicated effective inhibition against selected bacterial strains, highlighting the potential for developing new antimicrobial agents based on the biphenyl structure .
- Polymeric Applications : Research has demonstrated that BPDC can be utilized in synthesizing poly(aryl ether-bisketone)s with high molecular weights. These polymers exhibit desirable mechanical properties and thermal resistance, making them suitable for engineering applications .
- Metal-Organic Frameworks (MOFs) : Studies have shown that BPDC can serve as a linker in MOFs, which are used for catalysis and gas storage. The structural integrity and functionality of these frameworks can be tuned by altering the synthesis conditions, demonstrating BPDC's versatility in material science .
Properties
IUPAC Name |
4-(4-carboxyphenyl)benzoic acid | |
---|---|---|
Source | PubChem | |
URL | https://pubchem.ncbi.nlm.nih.gov | |
Description | Data deposited in or computed by PubChem | |
InChI |
InChI=1S/C14H10O4/c15-13(16)11-5-1-9(2-6-11)10-3-7-12(8-4-10)14(17)18/h1-8H,(H,15,16)(H,17,18) | |
Source | PubChem | |
URL | https://pubchem.ncbi.nlm.nih.gov | |
Description | Data deposited in or computed by PubChem | |
InChI Key |
NEQFBGHQPUXOFH-UHFFFAOYSA-N | |
Source | PubChem | |
URL | https://pubchem.ncbi.nlm.nih.gov | |
Description | Data deposited in or computed by PubChem | |
Canonical SMILES |
C1=CC(=CC=C1C2=CC=C(C=C2)C(=O)O)C(=O)O | |
Source | PubChem | |
URL | https://pubchem.ncbi.nlm.nih.gov | |
Description | Data deposited in or computed by PubChem | |
Molecular Formula |
C14H10O4 | |
Source | PubChem | |
URL | https://pubchem.ncbi.nlm.nih.gov | |
Description | Data deposited in or computed by PubChem | |
DSSTOX Substance ID |
DTXSID7061140 | |
Record name | [1,1'-Biphenyl]-4,4'-dicarboxylic acid | |
Source | EPA DSSTox | |
URL | https://comptox.epa.gov/dashboard/DTXSID7061140 | |
Description | DSSTox provides a high quality public chemistry resource for supporting improved predictive toxicology. | |
Molecular Weight |
242.23 g/mol | |
Source | PubChem | |
URL | https://pubchem.ncbi.nlm.nih.gov | |
Description | Data deposited in or computed by PubChem | |
Physical Description |
Coarse white powder; [3M MSDS] | |
Record name | 4,4'-Biphenyldicarboxylic acid | |
Source | Haz-Map, Information on Hazardous Chemicals and Occupational Diseases | |
URL | https://haz-map.com/Agents/19962 | |
Description | Haz-Map® is an occupational health database designed for health and safety professionals and for consumers seeking information about the adverse effects of workplace exposures to chemical and biological agents. | |
Explanation | Copyright (c) 2022 Haz-Map(R). All rights reserved. Unless otherwise indicated, all materials from Haz-Map are copyrighted by Haz-Map(R). No part of these materials, either text or image may be used for any purpose other than for personal use. Therefore, reproduction, modification, storage in a retrieval system or retransmission, in any form or by any means, electronic, mechanical or otherwise, for reasons other than personal use, is strictly prohibited without prior written permission. | |
CAS No. |
787-70-2, 84787-70-2 | |
Record name | [1,1′-Biphenyl]-4,4′-dicarboxylic acid | |
Source | CAS Common Chemistry | |
URL | https://commonchemistry.cas.org/detail?cas_rn=787-70-2 | |
Description | CAS Common Chemistry is an open community resource for accessing chemical information. Nearly 500,000 chemical substances from CAS REGISTRY cover areas of community interest, including common and frequently regulated chemicals, and those relevant to high school and undergraduate chemistry classes. This chemical information, curated by our expert scientists, is provided in alignment with our mission as a division of the American Chemical Society. | |
Explanation | The data from CAS Common Chemistry is provided under a CC-BY-NC 4.0 license, unless otherwise stated. | |
Record name | (1,1'-Biphenyl)-4,4'-dicarboxylic acid | |
Source | ChemIDplus | |
URL | https://pubchem.ncbi.nlm.nih.gov/substance/?source=chemidplus&sourceid=0000787702 | |
Description | ChemIDplus is a free, web search system that provides access to the structure and nomenclature authority files used for the identification of chemical substances cited in National Library of Medicine (NLM) databases, including the TOXNET system. | |
Record name | 787-70-2 | |
Source | DTP/NCI | |
URL | https://dtp.cancer.gov/dtpstandard/servlet/dwindex?searchtype=NSC&outputformat=html&searchlist=60016 | |
Description | The NCI Development Therapeutics Program (DTP) provides services and resources to the academic and private-sector research communities worldwide to facilitate the discovery and development of new cancer therapeutic agents. | |
Explanation | Unless otherwise indicated, all text within NCI products is free of copyright and may be reused without our permission. Credit the National Cancer Institute as the source. | |
Record name | [1,1'-Biphenyl]-4,4'-dicarboxylic acid | |
Source | EPA Chemicals under the TSCA | |
URL | https://www.epa.gov/chemicals-under-tsca | |
Description | EPA Chemicals under the Toxic Substances Control Act (TSCA) collection contains information on chemicals and their regulations under TSCA, including non-confidential content from the TSCA Chemical Substance Inventory and Chemical Data Reporting. | |
Record name | [1,1'-Biphenyl]-4,4'-dicarboxylic acid | |
Source | EPA DSSTox | |
URL | https://comptox.epa.gov/dashboard/DTXSID7061140 | |
Description | DSSTox provides a high quality public chemistry resource for supporting improved predictive toxicology. | |
Record name | Sandalwood, ext. | |
Source | European Chemicals Agency (ECHA) | |
URL | https://echa.europa.eu/substance-information/-/substanceinfo/100.076.437 | |
Description | The European Chemicals Agency (ECHA) is an agency of the European Union which is the driving force among regulatory authorities in implementing the EU's groundbreaking chemicals legislation for the benefit of human health and the environment as well as for innovation and competitiveness. | |
Explanation | Use of the information, documents and data from the ECHA website is subject to the terms and conditions of this Legal Notice, and subject to other binding limitations provided for under applicable law, the information, documents and data made available on the ECHA website may be reproduced, distributed and/or used, totally or in part, for non-commercial purposes provided that ECHA is acknowledged as the source: "Source: European Chemicals Agency, http://echa.europa.eu/". Such acknowledgement must be included in each copy of the material. ECHA permits and encourages organisations and individuals to create links to the ECHA website under the following cumulative conditions: Links can only be made to webpages that provide a link to the Legal Notice page. | |
Record name | [1,1'-biphenyl]-4,4'-dicarboxylic acid | |
Source | European Chemicals Agency (ECHA) | |
URL | https://echa.europa.eu/substance-information/-/substanceinfo/100.011.208 | |
Description | The European Chemicals Agency (ECHA) is an agency of the European Union which is the driving force among regulatory authorities in implementing the EU's groundbreaking chemicals legislation for the benefit of human health and the environment as well as for innovation and competitiveness. | |
Explanation | Use of the information, documents and data from the ECHA website is subject to the terms and conditions of this Legal Notice, and subject to other binding limitations provided for under applicable law, the information, documents and data made available on the ECHA website may be reproduced, distributed and/or used, totally or in part, for non-commercial purposes provided that ECHA is acknowledged as the source: "Source: European Chemicals Agency, http://echa.europa.eu/". Such acknowledgement must be included in each copy of the material. ECHA permits and encourages organisations and individuals to create links to the ECHA website under the following cumulative conditions: Links can only be made to webpages that provide a link to the Legal Notice page. | |
Record name | 4,4′-Biphenyldicarboxylic acid | |
Source | FDA Global Substance Registration System (GSRS) | |
URL | https://gsrs.ncats.nih.gov/ginas/app/beta/substances/5D8ABY24N2 | |
Description | The FDA Global Substance Registration System (GSRS) enables the efficient and accurate exchange of information on what substances are in regulated products. Instead of relying on names, which vary across regulatory domains, countries, and regions, the GSRS knowledge base makes it possible for substances to be defined by standardized, scientific descriptions. | |
Explanation | Unless otherwise noted, the contents of the FDA website (www.fda.gov), both text and graphics, are not copyrighted. They are in the public domain and may be republished, reprinted and otherwise used freely by anyone without the need to obtain permission from FDA. Credit to the U.S. Food and Drug Administration as the source is appreciated but not required. | |
Retrosynthesis Analysis
AI-Powered Synthesis Planning: Our tool employs the Template_relevance Pistachio, Template_relevance Bkms_metabolic, Template_relevance Pistachio_ringbreaker, Template_relevance Reaxys, Template_relevance Reaxys_biocatalysis model, leveraging a vast database of chemical reactions to predict feasible synthetic routes.
One-Step Synthesis Focus: Specifically designed for one-step synthesis, it provides concise and direct routes for your target compounds, streamlining the synthesis process.
Accurate Predictions: Utilizing the extensive PISTACHIO, BKMS_METABOLIC, PISTACHIO_RINGBREAKER, REAXYS, REAXYS_BIOCATALYSIS database, our tool offers high-accuracy predictions, reflecting the latest in chemical research and data.
Strategy Settings
Precursor scoring | Relevance Heuristic |
---|---|
Min. plausibility | 0.01 |
Model | Template_relevance |
Template Set | Pistachio/Bkms_metabolic/Pistachio_ringbreaker/Reaxys/Reaxys_biocatalysis |
Top-N result to add to graph | 6 |
Feasible Synthetic Routes
Q1: What is the molecular formula and weight of BPDC?
A1: BPDC has the molecular formula C14H10O4 and a molecular weight of 242.23 g/mol.
Q2: What spectroscopic data is available for characterizing BPDC?
A2: Researchers commonly use techniques like infrared (IR) spectroscopy, nuclear magnetic resonance (NMR) spectroscopy, and X-ray diffraction to characterize BPDC. IR spectroscopy identifies characteristic functional groups, such as carboxyl groups, while NMR provides information about the compound's structure and bonding. X-ray diffraction is crucial for determining the crystal structure of BPDC and its complexes. [, , , , , , , , ]
Q3: What materials is BPDC compatible with?
A4: BPDC is compatible with various metal ions, making it a popular choice for constructing MOFs. Researchers have synthesized BPDC-based MOFs with copper, cadmium, zinc, zirconium, and other metal ions. [, , , , , , , , ] Furthermore, it can interact with other organic molecules through hydrogen bonding and π-π interactions, enabling the formation of diverse supramolecular structures. [, , , ]
Q4: Does BPDC possess any inherent catalytic properties?
A5: While BPDC itself may not be a potent catalyst, incorporating it into MOF structures can impart catalytic properties. For instance, researchers have developed BPDC-based MOFs with Lewis acid catalytic activity for reactions like the Knoevenagel condensation. []
Q5: How is BPDC used in the synthesis of Metal-Organic Frameworks (MOFs)?
A6: BPDC acts as an organic linker in MOF synthesis due to its ability to coordinate with metal ions through its carboxylate groups. The rigid biphenyl core of BPDC contributes to the formation of porous framework structures. These BPDC-based MOFs have shown potential in gas adsorption, separation, catalysis, and sensing. [, , , , , ]
Q6: What are some notable applications of BPDC-based MOFs?
A7: BPDC-based MOFs have shown promise in various applications, including: * CO2 capture and separation: Due to their porous nature and tunable pore sizes, some BPDC-based MOFs exhibit high CO2 uptake capacities and selectivities, making them suitable for CO2 capture from flue gas or natural gas. [] * Catalysis: BPDC-based MOFs, often with incorporated metal catalytic centers, have been studied as heterogeneous catalysts for various organic reactions. [, , ] * Sensing: The luminescent properties of some BPDC-based MOFs change upon interaction with specific analytes, enabling their use as sensors. For example, researchers have investigated their potential for detecting pollutants like nitrobenzene. []
Q7: How is computational chemistry used in research on BPDC and its derivatives?
A8: Computational techniques, like Density Functional Theory (DFT) calculations, are essential for understanding the electronic properties, bonding, and reactivity of BPDC and its complexes. For example, DFT can be used to predict the adsorption behavior of different gases in BPDC-based MOFs or simulate the electronic structure of BPDC-metal complexes. [, , ]
Q8: How do modifications to the BPDC structure influence its properties and applications?
A9: Modifying the BPDC structure can significantly impact its properties: * Functionalization: Introducing different functional groups on the biphenyl core can alter its electronic properties, solubility, and interactions with guest molecules. For example, researchers have investigated BPDC derivatives containing amine, alkyne, azide, and nitro groups to tune the properties of MOFs for specific applications. [] * Linker length: Altering the length of the linker by using analogs like terephthalic acid or 4,4'-stilbenedicarboxylic acid can influence the pore size and structure of the resulting MOFs, impacting their gas adsorption and separation abilities. [, ]
Disclaimer and Information on In-Vitro Research Products
Please be aware that all articles and product information presented on BenchChem are intended solely for informational purposes. The products available for purchase on BenchChem are specifically designed for in-vitro studies, which are conducted outside of living organisms. In-vitro studies, derived from the Latin term "in glass," involve experiments performed in controlled laboratory settings using cells or tissues. It is important to note that these products are not categorized as medicines or drugs, and they have not received approval from the FDA for the prevention, treatment, or cure of any medical condition, ailment, or disease. We must emphasize that any form of bodily introduction of these products into humans or animals is strictly prohibited by law. It is essential to adhere to these guidelines to ensure compliance with legal and ethical standards in research and experimentation.