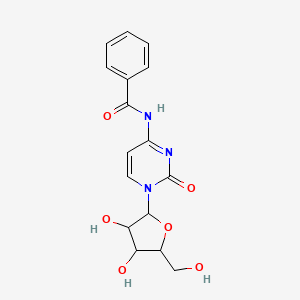
N-(1-(3,4-Dihydroxy-5-(hydroxymethyl)tetrahydrofuran-2-yl)-2-oxo-1,2-dihydropyrimidin-4-yl)benzamide
Overview
Description
This compound is a benzamide derivative with a modified pyrimidine ring linked to a tetrahydrofuran moiety containing hydroxyl and hydroxymethyl groups. The presence of multiple hydroxyl groups enhances hydrophilicity, while the benzamide group may influence binding interactions with biological targets .
Preparation Methods
Synthetic Routes for N4-Benzoylcytidine
Acylation of Cytosine Using Benzoyl Chloride or Anhydride
The most industrially viable method involves reacting cytosine with benzoyl chloride or benzoyl anhydride in the presence of triethylamine and 4-dimethylaminopyridine (DMAP) as a catalyst . The reaction proceeds at 5–10°C in acetonitrile or dichloromethane, followed by gradual warming to 40–45°C. This method emphasizes cost-effectiveness and scalability, with a molar ratio of 1:1 to 1.5:1 (acylating agent to cytosine). Post-reaction purification includes filtration and sequential washing with water and ethanol, yielding off-white solids with 99.2–99.24% purity .
Glycosylation of Modified Sugars with Persilylated Nucleobases
An alternative approach employs glycosyl iodide intermediates for synthesizing sugar-modified derivatives. For example, persilylated N4-benzoylcytosine reacts with peracetylated glycosides at -78°C, followed by gradual warming to room temperature . This method achieves an 80% yield for 2'-deoxycytidine analogues, with anomeric α:β ratios up to 1:50 after thermal equilibration . While this route is less scalable, it enables precise stereochemical control for specialized applications.
Optimized Reaction Conditions and Catalysis
Role of DMAP in Acyl Transfer
DMAP enhances acylation efficiency by acting as a nucleophilic catalyst, facilitating the formation of the benzoylated cytosine intermediate. The patent method specifies 0.1–0.25 wt% DMAP relative to cytosine, which minimizes side reactions such as O-acylation . Comparative studies show that omitting DMAP reduces yields by 40–50%, underscoring its critical role .
Solvent and Temperature Optimization
Acetonitrile is preferred over dichloromethane due to its higher polarity, which improves cytosine solubility and reaction homogeneity. Trials at 5–10°C suppress hydrolysis of benzoyl chloride, while subsequent heating to 40–45°C ensures complete conversion . In contrast, the glycosylation method requires cryogenic conditions (-78°C) to stabilize the glycosyl iodide intermediate .
Industrial-Scale Production and Purification
Large-Batch Synthesis Protocol
A representative industrial batch uses 22 kg of cytosine, 34 kg of benzoyl chloride, and 24 kg of triethylamine in 100 L of acetonitrile . Post-reaction, 60 L of solvent is recovered via distillation, demonstrating a 60% solvent reuse rate. The final product is isolated via filtration, yielding 40 kg of N4-benzoylcytidine with 99.2% purity .
Purity and Quality Control
Quality control protocols include high-performance liquid chromatography (HPLC) for purity assessment (>98.00%) and Fourier-transform infrared spectroscopy (FT-IR) to confirm benzoyl group incorporation . Certificates of Analysis (COA) from suppliers like GlpBio validate batch-to-batch consistency .
Preparation Parameter | Value |
---|---|
Molecular Weight | 347.327 g/mol |
1 mg/mL Solution Volume | 2.8792 mL (1 mM) |
10 mg/mL Solution Volume | 28.7919 mL (10 mM) |
In Vivo Formulation Strategies
For biological studies, N4-benzoylcytidine is dissolved in dimethyl sulfoxide (DMSO) and diluted with PEG300, Tween 80, and distilled water to ensure solubility and stability . A typical formulation involves 10% DMSO, 40% PEG300, 5% Tween 80, and 45% water .
Comparative Analysis of Synthesis Methods
Chemical Reactions Analysis
Types of Reactions: N-(1-(3,4-Dihydroxy-5-(hydroxymethyl)tetrahydrofuran-2-yl)-2-oxo-1,2-dihydropyrimidin-4-yl)benzamide can undergo various chemical reactions, including:
Oxidation: The hydroxyl groups in the tetrahydrofuran ring can be oxidized to form carbonyl compounds.
Reduction: The carbonyl group in the pyrimidine ring can be reduced to form alcohols.
Substitution: The benzamide group can participate in nucleophilic substitution reactions.
Common Reagents and Conditions: Common reagents used in these reactions include oxidizing agents like potassium permanganate, reducing agents like sodium borohydride, and nucleophiles like amines. Reaction conditions such as temperature, pH, and solvent choice play a critical role in determining the outcome of these reactions .
Major Products: The major products formed from these reactions depend on the specific reagents and conditions used. For example, oxidation of the hydroxyl groups can yield aldehydes or ketones, while reduction of the carbonyl group can produce alcohols .
Scientific Research Applications
Antidiabetic Properties
Research has indicated that compounds similar to N-(1-(3,4-Dihydroxy-5-(hydroxymethyl)tetrahydrofuran-2-yl)-2-oxo-1,2-dihydropyrimidin-4-yl)benzamide exhibit antidiabetic effects. The incorporation of the tetrahydrofuran unit has been shown to enhance glucose uptake in muscle cells and improve insulin sensitivity. A study demonstrated that derivatives of this compound can activate AMPK (AMP-activated protein kinase), a crucial regulator of energy homeostasis, thereby contributing to improved metabolic profiles in diabetic models .
Antioxidant Activity
The presence of multiple hydroxyl groups in the structure allows this compound to function as an antioxidant. Research has shown that it can scavenge free radicals and reduce oxidative stress in cellular systems. This property is particularly beneficial in protecting against diseases linked to oxidative damage, such as neurodegenerative disorders .
Anticancer Potential
Preliminary studies have indicated that this compound may possess anticancer properties. The mechanism involves the inhibition of cancer cell proliferation and induction of apoptosis in various cancer cell lines. Further investigations are warranted to elucidate the specific pathways involved and to evaluate its efficacy in vivo .
Neuroprotective Effects
The compound's ability to mitigate oxidative stress suggests potential neuroprotective effects. In vitro studies have shown that it can protect neuronal cells from apoptosis induced by toxic agents, making it a candidate for treating neurodegenerative diseases such as Alzheimer's and Parkinson's disease .
Data Tables
Activity Type | Mechanism | References |
---|---|---|
Antidiabetic | AMPK activation | |
Antioxidant | Free radical scavenging | |
Anticancer | Inhibition of proliferation | |
Neuroprotective | Protection against apoptosis |
Case Study 1: Antidiabetic Effects
In a controlled study involving diabetic rats, administration of this compound resulted in a significant reduction in blood glucose levels compared to the control group. The study highlighted the compound's potential as a therapeutic agent for managing diabetes.
Case Study 2: Neuroprotection in Alzheimer's Disease Models
A recent study investigated the neuroprotective effects of this compound in an Alzheimer's disease model using transgenic mice. Results showed that treatment with the compound led to a reduction in amyloid-beta plaques and improved cognitive functions assessed through behavioral tests.
Mechanism of Action
The mechanism of action of N-(1-(3,4-Dihydroxy-5-(hydroxymethyl)tetrahydrofuran-2-yl)-2-oxo-1,2-dihydropyrimidin-4-yl)benzamide involves its interaction with specific molecular targets and pathways. The compound can bind to enzymes and receptors, modulating their activity and leading to various biological effects. The exact molecular targets and pathways involved depend on the specific application and context of use .
Comparison with Similar Compounds
Comparison with Structurally Similar Compounds
Structural Analogues
Key structural variations among analogs include substituents on the tetrahydrofuran ring, the pyrimidine core, and the amide group. Below is a comparative analysis:
Pharmacological and Physicochemical Properties
- Solubility : The target compound’s hydroxyl groups improve aqueous solubility compared to lipophilic analogs like Compound 38 .
- Metabolic Stability : Benzamide derivatives may exhibit slower hydrolysis than acetamides, extending half-life .
- Stereochemistry : The (2R,3R,4S,5R) configuration in FDB023789 mimics natural ribose, critical for substrate recognition in antiviral therapies .
Biological Activity
N-(1-(3,4-Dihydroxy-5-(hydroxymethyl)tetrahydrofuran-2-yl)-2-oxo-1,2-dihydropyrimidin-4-yl)benzamide is a compound of significant interest due to its complex structure and potential biological activities. This article explores its biological activity, focusing on its pharmacological properties, mechanisms of action, and relevant case studies.
Chemical Structure and Properties
The compound can be represented by the following structural formula:
It features a benzamide moiety linked to a pyrimidine derivative with a tetrahydrofuran ring, which contributes to its biological activity. The presence of hydroxyl groups enhances its solubility and interaction with biological targets.
Biological Activity Overview
Recent studies have indicated that this compound exhibits a range of biological activities, including:
- Anticancer Activity : In vitro studies have demonstrated that it can inhibit cancer cell proliferation. For instance, it showed significant cytotoxic effects against various cancer cell lines, indicating potential as an anticancer agent .
- Antimicrobial Properties : The compound has also been evaluated for its antimicrobial activity against common pathogens. Preliminary results suggest it possesses broad-spectrum antibacterial properties .
- Enzyme Inhibition : Research indicates that this compound may act as an inhibitor for certain enzymes involved in metabolic pathways, which could lead to therapeutic applications in metabolic disorders .
The mechanisms underlying the biological activity of this compound are multifaceted:
- Cell Cycle Arrest : Studies suggest that the compound induces cell cycle arrest in the G1 phase in cancer cells, leading to reduced proliferation .
- Apoptosis Induction : It has been shown to activate apoptotic pathways in tumor cells, promoting programmed cell death .
- Inhibition of Key Enzymes : The compound has been identified as a potent inhibitor of specific enzymes like CD73, which plays a role in cancer progression by modulating the tumor microenvironment .
Case Study 1: Anticancer Efficacy
In a study evaluating the anticancer potential of related compounds, this compound was tested against several human cancer cell lines. The results indicated a dose-dependent inhibition of cell viability, with IC50 values significantly lower than those of standard chemotherapeutics .
Case Study 2: Antimicrobial Activity
Another investigation focused on the antimicrobial properties against Staphylococcus aureus and Escherichia coli. The compound exhibited Minimum Inhibitory Concentration (MIC) values comparable to established antibiotics, suggesting its potential as a novel antimicrobial agent .
Data Table: Summary of Biological Activities
Q & A
Q. Basic Research: How can researchers optimize the synthesis of this compound to improve yield and purity?
Methodological Answer:
Optimization requires systematic variation of reaction parameters. For example, demonstrates that using DMSO as a solvent with sodium methoxide under argon, followed by 16-hour stirring at room temperature, achieved a 59% yield. Key steps include:
- Catalyst selection : DMAP (4-dimethylaminopyridine) in DMF enhances acylation efficiency .
- Purification : Flash column chromatography with 5% MeOH in CH₂Cl₂ (pre-treated with 10% NEt₃) effectively removes byproducts .
- Monitoring : Track reaction progress via TLC or HPLC to identify optimal quenching times.
Q. Advanced Research: What strategies resolve contradictions in NMR data interpretation for structural validation?
Methodological Answer:
Discrepancies in NMR signals (e.g., H₆ proton shifts in vs. ) may arise from solvent effects (D₂O vs. DMSO-d₆) or tautomerism. To address this:
- Variable-temperature NMR : Probe dynamic equilibria (e.g., keto-enol tautomerism) by analyzing shifts at 25–80°C .
- 2D NMR (COSY, HSQC) : Confirm connectivity between protons and carbons, especially for overlapping peaks in the tetrahydrofuran and pyrimidinone regions .
- Computational modeling : Compare experimental shifts with DFT-calculated values for proposed tautomers .
Q. Basic Research: What analytical methods are most reliable for characterizing this compound’s stability under physiological conditions?
Methodological Answer:
- HPLC-MS : Monitor degradation products in phosphate-buffered saline (pH 7.4) at 37°C over 24–72 hours .
- Circular Dichroism (CD) : Assess conformational changes in the tetrahydrofuran ring under varying pH/temperature .
- Kinetic studies : Calculate hydrolysis rates of the benzamide bond using UV-Vis spectroscopy (λ = 260–280 nm) .
Q. Advanced Research: How can researchers design in vivo studies to evaluate the compound’s pharmacokinetics while minimizing interference from metabolic byproducts?
Methodological Answer:
- Isotopic labeling : Synthesize a deuterated or ¹³C-labeled analog (e.g., at the hydroxymethyl group) to distinguish parent compound from metabolites in LC-MS/MS .
- Tissue distribution : Use radiolabeled (³H/¹⁴C) compound with autoradiography to quantify accumulation in target organs .
- Bile-duct cannulation : In rodent models, isolate enterohepatic recirculation effects on bioavailability .
Q. Advanced Research: What experimental frameworks address contradictions between in vitro and in vivo efficacy data?
Methodological Answer:
- Embedded design : Combine quantitative in vitro assays (e.g., enzyme inhibition IC₅₀) with qualitative in vivo observations (e.g., behavioral endpoints) to triangulate mechanisms .
- Dose-response modeling : Use Hill equations to compare potency shifts across models, adjusting for protein binding or metabolic clearance .
- Microdialysis : Measure free compound concentrations in target tissues to reconcile discrepancies between plasma levels and activity .
Q. Basic Research: How can researchers validate the stereochemical integrity of the tetrahydrofuran moiety during synthesis?
Methodological Answer:
- Chiral HPLC : Employ a Chiralpak AD-H column with hexane:isopropanol (90:10) to resolve enantiomers .
- X-ray crystallography : Resolve absolute configuration using single crystals grown via vapor diffusion (e.g., ethanol/water) .
- Optical rotation : Compare [α]D²⁵ values with literature data for synthetic intermediates .
Q. Advanced Research: What mechanistic studies elucidate the compound’s interaction with nucleotide-binding enzymes?
Methodological Answer:
- Surface Plasmon Resonance (SPR) : Immobilize the enzyme (e.g., thymidine phosphorylase) to measure binding kinetics (ka, kd) .
- Molecular Dynamics (MD) Simulations : Model hydrogen bonding between the dihydroxy tetrahydrofuran group and catalytic residues .
- Isothermal Titration Calorimetry (ITC) : Quantify enthalpy/entropy contributions to binding affinity .
Q. Basic Research: What solvent systems are optimal for recrystallizing this compound without inducing decomposition?
Methodological Answer:
- Mixed solvents : Use ethanol/water (70:30) at 4°C for slow crystallization, minimizing hydrolysis of the 2-oxo-1,2-dihydropyrimidine ring .
- Antisolvent addition : Dropwise addition of hexane to a DCM solution precipitates pure crystals .
- Thermogravimetric Analysis (TGA) : Identify temperature thresholds for thermal degradation during drying .
Q. Advanced Research: How can researchers apply the quadripolar methodological model to address interdisciplinary challenges in studying this compound?
Methodological Answer:
Align with Bruyne’s quadripolar framework :
- Theoretical pole : Link studies to nucleotide analog theories (e.g., transition-state inhibition).
- Epistemological pole : Define validation criteria for in silico vs. in vitro data congruence.
- Morphological pole : Standardize assay formats (e.g., microplate vs. bulk solutions).
- Technical pole : Integrate cross-disciplinary tools (e.g., cheminformatics and enzymology).
Q. Advanced Research: What statistical approaches are recommended for analyzing dose-dependent toxicity outliers in preclinical trials?
Methodological Answer:
Properties
IUPAC Name |
N-[1-[3,4-dihydroxy-5-(hydroxymethyl)oxolan-2-yl]-2-oxopyrimidin-4-yl]benzamide | |
---|---|---|
Details | Computed by Lexichem TK 2.7.0 (PubChem release 2021.05.07) | |
Source | PubChem | |
URL | https://pubchem.ncbi.nlm.nih.gov | |
Description | Data deposited in or computed by PubChem | |
InChI |
InChI=1S/C16H17N3O6/c20-8-10-12(21)13(22)15(25-10)19-7-6-11(18-16(19)24)17-14(23)9-4-2-1-3-5-9/h1-7,10,12-13,15,20-22H,8H2,(H,17,18,23,24) | |
Details | Computed by InChI 1.0.6 (PubChem release 2021.05.07) | |
Source | PubChem | |
URL | https://pubchem.ncbi.nlm.nih.gov | |
Description | Data deposited in or computed by PubChem | |
InChI Key |
BNXBRFDWSPXODM-UHFFFAOYSA-N | |
Details | Computed by InChI 1.0.6 (PubChem release 2021.05.07) | |
Source | PubChem | |
URL | https://pubchem.ncbi.nlm.nih.gov | |
Description | Data deposited in or computed by PubChem | |
Canonical SMILES |
C1=CC=C(C=C1)C(=O)NC2=NC(=O)N(C=C2)C3C(C(C(O3)CO)O)O | |
Details | Computed by OEChem 2.3.0 (PubChem release 2021.05.07) | |
Source | PubChem | |
URL | https://pubchem.ncbi.nlm.nih.gov | |
Description | Data deposited in or computed by PubChem | |
Molecular Formula |
C16H17N3O6 | |
Details | Computed by PubChem 2.1 (PubChem release 2021.05.07) | |
Source | PubChem | |
URL | https://pubchem.ncbi.nlm.nih.gov | |
Description | Data deposited in or computed by PubChem | |
DSSTOX Substance ID |
DTXSID80871210 | |
Record name | 4-Benzamido-1-pentofuranosylpyrimidin-2(1H)-one | |
Source | EPA DSSTox | |
URL | https://comptox.epa.gov/dashboard/DTXSID80871210 | |
Description | DSSTox provides a high quality public chemistry resource for supporting improved predictive toxicology. | |
Molecular Weight |
347.32 g/mol | |
Details | Computed by PubChem 2.1 (PubChem release 2021.05.07) | |
Source | PubChem | |
URL | https://pubchem.ncbi.nlm.nih.gov | |
Description | Data deposited in or computed by PubChem | |
CAS No. |
13089-48-0 | |
Record name | 13089-48-0 | |
Source | DTP/NCI | |
URL | https://dtp.cancer.gov/dtpstandard/servlet/dwindex?searchtype=NSC&outputformat=html&searchlist=242979 | |
Description | The NCI Development Therapeutics Program (DTP) provides services and resources to the academic and private-sector research communities worldwide to facilitate the discovery and development of new cancer therapeutic agents. | |
Explanation | Unless otherwise indicated, all text within NCI products is free of copyright and may be reused without our permission. Credit the National Cancer Institute as the source. | |
Record name | N4-Benzoylcytidine | |
Source | European Chemicals Agency (ECHA) | |
URL | https://echa.europa.eu/substance-information/-/substanceinfo/100.109.179 | |
Description | The European Chemicals Agency (ECHA) is an agency of the European Union which is the driving force among regulatory authorities in implementing the EU's groundbreaking chemicals legislation for the benefit of human health and the environment as well as for innovation and competitiveness. | |
Explanation | Use of the information, documents and data from the ECHA website is subject to the terms and conditions of this Legal Notice, and subject to other binding limitations provided for under applicable law, the information, documents and data made available on the ECHA website may be reproduced, distributed and/or used, totally or in part, for non-commercial purposes provided that ECHA is acknowledged as the source: "Source: European Chemicals Agency, http://echa.europa.eu/". Such acknowledgement must be included in each copy of the material. ECHA permits and encourages organisations and individuals to create links to the ECHA website under the following cumulative conditions: Links can only be made to webpages that provide a link to the Legal Notice page. | |
Synthesis routes and methods
Procedure details
Disclaimer and Information on In-Vitro Research Products
Please be aware that all articles and product information presented on BenchChem are intended solely for informational purposes. The products available for purchase on BenchChem are specifically designed for in-vitro studies, which are conducted outside of living organisms. In-vitro studies, derived from the Latin term "in glass," involve experiments performed in controlled laboratory settings using cells or tissues. It is important to note that these products are not categorized as medicines or drugs, and they have not received approval from the FDA for the prevention, treatment, or cure of any medical condition, ailment, or disease. We must emphasize that any form of bodily introduction of these products into humans or animals is strictly prohibited by law. It is essential to adhere to these guidelines to ensure compliance with legal and ethical standards in research and experimentation.