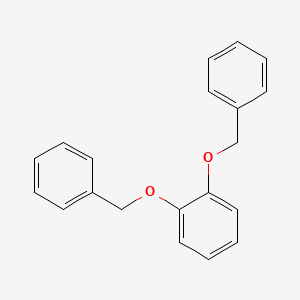
1,2-Bis(benzyloxy)benzene
Overview
Description
1,2-Bis(benzyloxy)benzene is an organic compound with the molecular formula C20H18O2. It consists of a benzene ring substituted with two benzyloxy groups at the 1 and 2 positions. This compound is part of the larger family of benzene derivatives, which are known for their aromatic properties and wide range of applications in various fields.
Preparation Methods
Synthetic Routes and Reaction Conditions
1,2-Bis(benzyloxy)benzene can be synthesized through several methods:
Hydrogenation: This process involves the hydrogenation of this compound to produce 1,2-bis(benzyl)benzene.
Nucleophilic Substitution: The benzyloxy groups on this compound can be replaced by nucleophiles in a substitution reaction.
Acid-Catalyzed Hydrolysis: This method involves the hydrolysis of this compound in the presence of an acid (e.g., concentrated sulfuric acid) to produce 1,2-benzenediol (catechol) and benzyl alcohol.
Industrial Production Methods
Industrial production methods for this compound typically involve large-scale synthesis using the above-mentioned routes, with optimization for yield and purity. The choice of method depends on the desired application and the availability of reagents.
Chemical Reactions Analysis
Types of Reactions
1,2-Bis(benzyloxy)benzene undergoes various chemical reactions, including:
Oxidation: This compound can be oxidized to form various products depending on the reaction conditions.
Substitution: The benzyloxy groups can be replaced by nucleophiles in substitution reactions.
Common Reagents and Conditions
Oxidizing Agents: Strong oxidizing agents such as potassium permanganate (KMnO4) or chromium trioxide (CrO3) are commonly used for oxidation reactions.
Reducing Agents: Metal catalysts like palladium or platinum, along with hydrogen gas, are used for reduction reactions.
Nucleophiles: Strong nucleophiles like sodium methoxide (NaOMe) are used for substitution reactions.
Major Products
Oxidation: Benzoquinone derivatives.
Reduction: 1,2-Bis(benzyl)benzene or the corresponding diol.
Substitution: Sodium 1,2-bis(methoxy)benzene and benzyl alcohol.
Scientific Research Applications
1,2-Bis(benzyloxy)benzene has several applications in scientific research:
Mechanism of Action
The mechanism of action of 1,2-bis(benzyloxy)benzene involves its interaction with various molecular targets and pathways. The benzyloxy groups can undergo nucleophilic substitution, oxidation, and reduction reactions, leading to the formation of different products. These reactions are facilitated by the aromatic nature of the benzene ring, which stabilizes intermediates and transition states .
Comparison with Similar Compounds
1,2-Bis(benzyloxy)benzene can be compared with other similar compounds, such as:
1,2-Bis(benzyl)benzene: Formed by the hydrogenation of this compound.
1,2-Benzenediol (catechol): Formed by the acid-catalyzed hydrolysis of this compound.
1,2-Bis(methoxy)benzene: Formed by nucleophilic substitution of the benzyloxy groups with methoxy groups.
These compounds share similar structural features but differ in their functional groups and reactivity, highlighting the versatility and uniqueness of this compound .
Properties
IUPAC Name |
1,2-bis(phenylmethoxy)benzene | |
---|---|---|
Details | Computed by Lexichem TK 2.7.0 (PubChem release 2021.05.07) | |
Source | PubChem | |
URL | https://pubchem.ncbi.nlm.nih.gov | |
Description | Data deposited in or computed by PubChem | |
InChI |
InChI=1S/C20H18O2/c1-3-9-17(10-4-1)15-21-19-13-7-8-14-20(19)22-16-18-11-5-2-6-12-18/h1-14H,15-16H2 | |
Details | Computed by InChI 1.0.6 (PubChem release 2021.05.07) | |
Source | PubChem | |
URL | https://pubchem.ncbi.nlm.nih.gov | |
Description | Data deposited in or computed by PubChem | |
InChI Key |
NGAVLQZEBCIHII-UHFFFAOYSA-N | |
Details | Computed by InChI 1.0.6 (PubChem release 2021.05.07) | |
Source | PubChem | |
URL | https://pubchem.ncbi.nlm.nih.gov | |
Description | Data deposited in or computed by PubChem | |
Canonical SMILES |
C1=CC=C(C=C1)COC2=CC=CC=C2OCC3=CC=CC=C3 | |
Details | Computed by OEChem 2.3.0 (PubChem release 2021.05.07) | |
Source | PubChem | |
URL | https://pubchem.ncbi.nlm.nih.gov | |
Description | Data deposited in or computed by PubChem | |
Molecular Formula |
C20H18O2 | |
Details | Computed by PubChem 2.1 (PubChem release 2021.05.07) | |
Source | PubChem | |
URL | https://pubchem.ncbi.nlm.nih.gov | |
Description | Data deposited in or computed by PubChem | |
Molecular Weight |
290.4 g/mol | |
Details | Computed by PubChem 2.1 (PubChem release 2021.05.07) | |
Source | PubChem | |
URL | https://pubchem.ncbi.nlm.nih.gov | |
Description | Data deposited in or computed by PubChem | |
Synthesis routes and methods I
Procedure details
Synthesis routes and methods II
Procedure details
Disclaimer and Information on In-Vitro Research Products
Please be aware that all articles and product information presented on BenchChem are intended solely for informational purposes. The products available for purchase on BenchChem are specifically designed for in-vitro studies, which are conducted outside of living organisms. In-vitro studies, derived from the Latin term "in glass," involve experiments performed in controlled laboratory settings using cells or tissues. It is important to note that these products are not categorized as medicines or drugs, and they have not received approval from the FDA for the prevention, treatment, or cure of any medical condition, ailment, or disease. We must emphasize that any form of bodily introduction of these products into humans or animals is strictly prohibited by law. It is essential to adhere to these guidelines to ensure compliance with legal and ethical standards in research and experimentation.