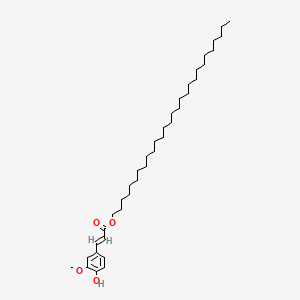
Octacosyl (E)-ferulate
Overview
Description
Octacosyl (E)-ferulate (CAS: 101959-37-9) is a long-chain alkyl ester of ferulic acid, with the molecular formula C₃₈H₆₆O₄ and a molecular weight of 586.93 g/mol . Structurally, it consists of a trans(E)-configured ferulic acid moiety (3-methoxy-4-hydroxycinnamic acid) esterified to a 28-carbon (octacosyl) alcohol chain. This compound is classified as a phenylpropanoid derivative and is notable for its lipophilic nature, which facilitates its integration into plant cuticular waxes and membranes .
Preparation Methods
Synthetic Routes and Reaction Conditions
The synthesis of octacosyl (E)-ferulate typically involves the esterification of octacosanol with ferulic acid. This reaction can be catalyzed by acidic or enzymatic catalysts. The reaction conditions often include:
Temperature: Typically around 60-80°C.
Catalysts: Acidic catalysts like sulfuric acid or enzymatic catalysts like lipases.
Solvents: Organic solvents such as toluene or hexane are commonly used to dissolve the reactants and facilitate the reaction.
Industrial Production Methods
In an industrial setting, the production of this compound may involve continuous flow reactors to ensure consistent product quality and yield. The use of biocatalysts, such as immobilized enzymes, can enhance the efficiency and selectivity of the esterification process.
Chemical Reactions Analysis
Oxidation Reactions
The compound undergoes oxidation at its phenolic and conjugated double-bond moieties:
-
Reagents : Potassium permanganate (KMnO₄) or hydrogen peroxide (H₂O₂).
-
Products : Quinones and oxidized ferulic acid derivatives, which retain antioxidant properties .
Reduction Reactions
Reduction targets the ester and double-bond functionalities:
-
Reagents : Lithium aluminum hydride (LiAlH₄).
-
Products : Octacosanol and reduced ferulic acid derivatives (e.g., dihydroferulic acid).
Substitution Reactions
Nucleophilic substitution occurs at the ester group:
-
Reagents : Sodium hydroxide (NaOH) or other nucleophiles.
-
Products : Alkoxyl or aminol derivatives, depending on the nucleophile used .
Antioxidant Activity and Radical Scavenging
Octacosyl (E)-ferulate demonstrates significant antioxidant capacity via radical scavenging:
DPPH Radical Scavenging
Compound | IC₅₀ (µg/mL) | Source |
---|---|---|
Ferulic acid | 15.61 ± 1.82 | |
This compound | >100 |
FRAP Assay
-
Trend : Antioxidant capacity decreases with increasing alkyl chain length (C5 cutoff) .
-
Mechanism : Electron donation from the phenolic hydroxyl group stabilizes radicals .
Enzymatic Transformations
-
Hydrolysis : Esterases cleave the ester bond, releasing ferulic acid and octacosanol .
-
Thermal degradation : At 220°C, the compound reduces thiobarbituric acid reactive species (TBARS) by 6-fold compared to controls .
Key Findings
-
Synthetic flexibility : Multiple methods (e.g., mixed anhydride, acid-catalyzed) enable scalable production .
-
Structure-reactivity relationship : Longer alkyl chains diminish radical scavenging but enhance thermal stability .
-
Biological relevance : Enzymatic hydrolysis releases bioactive ferulic acid, linking chemical stability to pharmacological effects .
Scientific Research Applications
Biomedical Applications
1.1 Antioxidant Properties
Octacosyl (E)-ferulate exhibits significant antioxidant activity, which is crucial for protecting cells from oxidative stress. Studies have shown that alkyl ferulates, including octacosyl ferulate, can scavenge free radicals effectively. For instance, the DPPH scavenging activity of various O-alkyl ferulates was evaluated, revealing that octacosyl ferulate has an IC50 value comparable to other potent antioxidants .
Table 1: Antioxidant Activity of O-Alkyl Ferulates
Compound | IC50 (µg/mL) |
---|---|
Octacosyl Ferulate | TBD |
Dodecyl Ferulate | 56.50 ± 2.38 |
Hexadecyl Ferulate | 90.43 ± 1.63 |
1.2 Anti-inflammatory Effects
Research indicates that this compound can modulate inflammatory responses. In vitro studies demonstrated its ability to inhibit nitric oxide production in macrophages, suggesting potential applications in treating inflammatory diseases .
Case Study: Inhibition of Nitric Oxide Production
- Objective: Assess the anti-inflammatory effects of this compound.
- Method: Macrophage cell lines were treated with varying concentrations of this compound.
- Results: Significant reduction in nitric oxide levels was observed at concentrations above 10 µM.
Food Science Applications
2.1 Natural Preservative
Due to its antioxidant properties, this compound is being investigated as a natural preservative in food products. Its effectiveness in preventing lipid oxidation in oils has been documented, making it a candidate for enhancing the shelf life of fatty foods .
Table 2: Effectiveness of Octacosyl Ferulate as a Food Preservative
Food Matrix | Oxidation Rate Reduction (%) |
---|---|
Fish Oil-enriched Mayonnaise | TBD |
Sunflower Oil | TBD |
2.2 Enhancement of Nutritional Quality
The incorporation of this compound into food formulations has been shown to improve the nutritional profile by enhancing the stability of polyunsaturated fatty acids (PUFAs), which are susceptible to oxidation during storage .
Material Science Applications
3.1 Development of Functional Materials
This compound is also being explored for its potential in developing functional materials such as biodegradable films and coatings. Its lipophilic nature allows it to form stable emulsions and films that can be used for packaging applications .
Case Study: Biodegradable Film Formation
- Objective: Evaluate the feasibility of using this compound in biodegradable films.
- Method: Films were prepared using this compound as a plasticizer.
- Results: The films exhibited good mechanical properties and moisture barrier capabilities.
Mechanism of Action
The mechanism of action of octacosyl (E)-ferulate primarily involves its antioxidant activity. The phenolic group in ferulic acid can donate hydrogen atoms to neutralize free radicals, thereby preventing oxidative damage to cells and tissues. Additionally, the long-chain fatty alcohol (octacosanol) may enhance the compound’s ability to integrate into cell membranes, providing further protection against oxidative stress.
Comparison with Similar Compounds
Key Characteristics:
- Natural Sources : Isolated from Rhodiola henryi (菱叶红景天) , Morus spp. (mulberry) , and Spiraea japonica .
- Biological Activities :
Octacosyl (E)-ferulate belongs to a broader class of alkyl ferulates , characterized by varying chain lengths and functional group configurations. Below is a systematic comparison with structurally and functionally related compounds:
Structural Analogues: Alkyl Ferulates
Table 1: Key Alkyl Ferulates and Their Properties
Key Observations:
Chain Length and Hydrophobicity :
- Longer alkyl chains (e.g., C28 in octacosyl vs. C20 in eicosyl ferulate) enhance hydrophobicity, critical for forming water-repellent plant surfaces . This compound’s C28 chain optimizes membrane integration, whereas shorter-chain analogues like eicosyl ferulate exhibit reduced lipid solubility .
- Enzymatic synthesis involves acyltransferases (e.g., AWATs/WSD1) for esterification, a mechanism conserved across alkyl ferulates .
Hexacosyl (E)-ferulate, however, shows unique antioxidant activity in Echinops spp., suggesting chain length influences redox modulation .
Isomerism :
- The trans(E)-configuration of octacosyl ferulate is thermodynamically stable compared to cis(Z)-isomers, which exhibit distinct NMR profiles (e.g., coupling constants: 16.1 Hz for trans vs. 12.8 Hz for cis) . Cis-isomers are rarely observed in natural extracts but may form during storage .
Functional Analogues: Non-Ferulate Lipophilic Esters
Table 2: Comparison with Non-Ferulate Esters
Key Differences:
- Biosynthetic Pathways: Ferulates require phenylpropanoid pathway intermediates, whereas acetate esters derive from fatty acid metabolism .
Biological Activity
Octacosyl (E)-ferulate, a long-chain fatty acid ester derived from ferulic acid and octacosanol, has garnered attention for its diverse biological activities. This compound is primarily extracted from plants such as Erythrina caffra and exhibits a range of pharmacological properties, making it a subject of interest in various fields including medicine, pharmacology, and cosmetics.
- Molecular Formula: CHO
- Molecular Weight: 586.93 g/mol
- Density: 0.9 ± 0.1 g/cm³
- Boiling Point: 659.1 ± 40.0 °C at 760 mmHg
- LogP: 15.75
These properties contribute to its lipophilicity, enhancing its integration into lipid membranes, which is particularly beneficial in skincare formulations.
Target of Action:
this compound acts on several biological pathways:
- Cholesterol-lowering Effects: It has been shown to reduce cholesterol levels, potentially benefiting cardiovascular health.
- Antiaggregatory Properties: The compound exhibits antiaggregatory effects, which may help in preventing thrombosis.
- Cytoprotective Effects: It provides protection to cells against oxidative stress and inflammation.
- Ergogenic Properties: It is noted for enhancing physical performance, making it relevant in sports nutrition.
Biochemical Pathways:
Research indicates that this compound influences mitochondrial function and energy metabolism through oxidative phosphorylation pathways. This is crucial for understanding its role in bioenergetics and cellular respiration.
Antimicrobial Activity
This compound demonstrates significant antimicrobial properties:
- Against Bacteria: It has shown antibacterial activity against Staphylococcus aureus, with a minimum inhibitory concentration (MIC) of 0.125 mg/mL. Weaker activity was observed against other strains like Pseudomonas aeruginosa and Escherichia coli .
Anticancer Activity
Research indicates that this compound exhibits inhibitory effects on tumor promotion:
- Mechanism: It suppresses protein kinase C (PK-C) activity, which is involved in tumorigenesis. This suggests potential applications in cancer prevention strategies .
Antiplasmodial Activity
Studies have identified this compound as an antiplasmodial agent:
- Source: Isolated from the root bark of Erythrina abyssinica, it has shown effectiveness against malaria parasites .
Comparative Analysis with Similar Compounds
Compound | Structure | Biological Activity |
---|---|---|
Octacosyl Ferulate | CHO | Antimicrobial, anticancer, antiplasmodial |
Octyl Ferulate | CHO | Antioxidant, anti-inflammatory |
Ethyl Ferulate | CHO | Moderate antimicrobial |
This compound is distinguished by its longer carbon chain, which enhances its lipophilicity compared to shorter-chain esters like octyl and ethyl ferulates.
Case Studies and Research Findings
-
Cholesterol-Lowering Effects:
A study demonstrated that this compound significantly reduced serum cholesterol levels in animal models, indicating its potential as a dietary supplement for cardiovascular health. -
Antimicrobial Efficacy:
In vitro studies revealed that this compound effectively inhibited the growth of various bacterial strains, suggesting its application in developing new antimicrobial agents . -
Tumor Promotion Inhibition:
Research conducted on mouse models showed that administration of this compound resulted in reduced tumor formation when exposed to carcinogenic agents .
Q & A
Basic Research Questions
Q. How can Octacosyl (E)-ferulate be isolated and purified from natural sources?
- Methodological Answer : Isolation typically involves solvent extraction (e.g., ethanol or methanol) followed by chromatographic techniques such as column chromatography or preparative HPLC. For example, this compound has been isolated from Bauhinia racemosa using silica gel chromatography and verified via NMR and mass spectrometry . Key challenges include separating it from structurally similar compounds like β-sitosteryl ferulate, requiring gradient elution with hexane-ethyl acetate mixtures .
Q. What analytical techniques are suitable for quantifying this compound in complex matrices?
- Methodological Answer : Reverse-phase HPLC with UV detection (280–320 nm) is widely used, especially for lipid-rich samples. For instance, studies on fish oil-enriched milk employed C18 columns with acetonitrile-water gradients to resolve this compound from other ferulate esters (e.g., methyl or ethyl ferulates) . Confirmation of identity should combine retention time matching with high-resolution mass spectrometry (HRMS) or FTIR to distinguish it from isomers like hexacosyl ferulate .
Q. How can researchers assess the stability of this compound under experimental conditions?
- Methodological Answer : Stability can be evaluated by monitoring degradation products (e.g., free ferulic acid) via HPLC over time under varying pH, temperature, and oxidative stress. For lipid systems, accelerated storage trials (e.g., 5°C for 13 days) with periodic sampling are effective. Oxidation markers like 1-penten-3-one and 2,4-heptadienal should be quantified to correlate stability with antioxidant efficacy .
Advanced Research Questions
Q. What experimental designs are optimal for studying this compound’s antioxidant mechanism in multiphase systems?
- Methodological Answer : Partitioning studies (e.g., oil/emulsion, aqueous, precipitate phases) are critical. In fish oil-enriched milk, this compound’s long alkyl chain localizes it predominantly in the oil phase, requiring phase-specific sampling (e.g., centrifugation followed by solvent extraction) to assess interfacial activity . Couple this with radical scavenging assays (DPPH/ABTS) in each phase to map antioxidant contribution spatially .
Q. How does alkyl chain length influence the efficacy of ferulate esters like this compound?
- Methodological Answer : Comparative studies using ferulates with varying chain lengths (C1–C28) reveal that longer chains (e.g., C28) enhance lipid-phase solubility but reduce interfacial concentration, limiting access to aqueous-phase radicals. For example, dodecyl ferulate (C12) showed higher interfacial activity but lower oxidative stability than this compound in lipid emulsions . Use Langmuir trough experiments to quantify surface pressure and molecular packing .
Q. How can researchers resolve contradictions in this compound’s reported bioactivity across studies?
- Methodological Answer : Discrepancies often arise from differences in assay systems (e.g., in vitro vs. in vivo) or sample purity. For anti-inflammatory activity, validate findings using multiple models:
- In vitro : PK-C inhibition assays (IC50 determination via kinase activity kits) .
- In vivo : TPA-induced mouse ear edema models with dose-response curves and histological analysis .
Ensure compound purity (>95% by HPLC) and control for co-extracted phytochemicals (e.g., lupeol) that may synergize or antagonize effects .
Q. What strategies mitigate interference from matrix effects when analyzing this compound in plant extracts?
- Methodological Answer : Matrix-matched calibration and standard addition methods are essential. For example, spiking plant extracts with known concentrations of this compound and comparing recovery rates (80–120%) can identify matrix-induced suppression/enhancement. Solid-phase extraction (SPE) with C18 cartridges effectively removes polar interferents like flavonoids .
Q. Data Analysis and Interpretation
Q. How should researchers interpret conflicting data on this compound’s phase-specific antioxidant behavior?
- Methodological Answer : Contradictions may stem from differing lipid compositions (e.g., milk vs. bulk oil). Use multivariate analysis (e.g., PCA) to correlate phase distribution data (Figure 8 ) with antioxidant metrics. For instance, in systems with high interfacial area, shorter-chain ferulates outperform this compound, while bulk oil systems favor longer-chain analogs .
Q. What statistical approaches are recommended for dose-response studies involving this compound?
- Methodological Answer : Nonlinear regression (e.g., log-dose vs. response) with software like GraphPad Prism is standard. For anti-inflammatory assays, calculate EC50 values using four-parameter logistic curves. Report confidence intervals and perform outlier tests (e.g., Grubbs’ test) to ensure robustness, especially in heterogeneous biological systems .
Q. Synthesis and Modification
Q. What synthetic routes are available for this compound, and how can its bioavailability be improved?
- Methodological Answer : Chemical synthesis involves esterification of ferulic acid with octacosanol using DCC/DMAP coupling. To enhance bioavailability, nanoemulsion formulations (e.g., Tween 80/lecithin) increase aqueous dispersibility. Alternatively, synthesize glycosylated derivatives to improve intestinal absorption, as demonstrated for similar polyphenol esters .
Properties
IUPAC Name |
octacosyl (E)-3-(4-hydroxy-3-methoxyphenyl)prop-2-enoate | |
---|---|---|
Source | PubChem | |
URL | https://pubchem.ncbi.nlm.nih.gov | |
Description | Data deposited in or computed by PubChem | |
InChI |
InChI=1S/C38H66O4/c1-3-4-5-6-7-8-9-10-11-12-13-14-15-16-17-18-19-20-21-22-23-24-25-26-27-28-33-42-38(40)32-30-35-29-31-36(39)37(34-35)41-2/h29-32,34,39H,3-28,33H2,1-2H3/b32-30+ | |
Source | PubChem | |
URL | https://pubchem.ncbi.nlm.nih.gov | |
Description | Data deposited in or computed by PubChem | |
InChI Key |
PIGLOISSVVAGBD-NHQGMKOOSA-N | |
Source | PubChem | |
URL | https://pubchem.ncbi.nlm.nih.gov | |
Description | Data deposited in or computed by PubChem | |
Canonical SMILES |
CCCCCCCCCCCCCCCCCCCCCCCCCCCCOC(=O)C=CC1=CC(=C(C=C1)O)OC | |
Source | PubChem | |
URL | https://pubchem.ncbi.nlm.nih.gov | |
Description | Data deposited in or computed by PubChem | |
Isomeric SMILES |
CCCCCCCCCCCCCCCCCCCCCCCCCCCCOC(=O)/C=C/C1=CC(=C(C=C1)O)OC | |
Source | PubChem | |
URL | https://pubchem.ncbi.nlm.nih.gov | |
Description | Data deposited in or computed by PubChem | |
Molecular Formula |
C38H66O4 | |
Source | PubChem | |
URL | https://pubchem.ncbi.nlm.nih.gov | |
Description | Data deposited in or computed by PubChem | |
Molecular Weight |
586.9 g/mol | |
Source | PubChem | |
URL | https://pubchem.ncbi.nlm.nih.gov | |
Description | Data deposited in or computed by PubChem | |
CAS No. |
35321-71-2 | |
Record name | Cluytyl ferulate | |
Source | ChemIDplus | |
URL | https://pubchem.ncbi.nlm.nih.gov/substance/?source=chemidplus&sourceid=0035321712 | |
Description | ChemIDplus is a free, web search system that provides access to the structure and nomenclature authority files used for the identification of chemical substances cited in National Library of Medicine (NLM) databases, including the TOXNET system. | |
Retrosynthesis Analysis
AI-Powered Synthesis Planning: Our tool employs the Template_relevance Pistachio, Template_relevance Bkms_metabolic, Template_relevance Pistachio_ringbreaker, Template_relevance Reaxys, Template_relevance Reaxys_biocatalysis model, leveraging a vast database of chemical reactions to predict feasible synthetic routes.
One-Step Synthesis Focus: Specifically designed for one-step synthesis, it provides concise and direct routes for your target compounds, streamlining the synthesis process.
Accurate Predictions: Utilizing the extensive PISTACHIO, BKMS_METABOLIC, PISTACHIO_RINGBREAKER, REAXYS, REAXYS_BIOCATALYSIS database, our tool offers high-accuracy predictions, reflecting the latest in chemical research and data.
Strategy Settings
Precursor scoring | Relevance Heuristic |
---|---|
Min. plausibility | 0.01 |
Model | Template_relevance |
Template Set | Pistachio/Bkms_metabolic/Pistachio_ringbreaker/Reaxys/Reaxys_biocatalysis |
Top-N result to add to graph | 6 |
Feasible Synthetic Routes
Disclaimer and Information on In-Vitro Research Products
Please be aware that all articles and product information presented on BenchChem are intended solely for informational purposes. The products available for purchase on BenchChem are specifically designed for in-vitro studies, which are conducted outside of living organisms. In-vitro studies, derived from the Latin term "in glass," involve experiments performed in controlled laboratory settings using cells or tissues. It is important to note that these products are not categorized as medicines or drugs, and they have not received approval from the FDA for the prevention, treatment, or cure of any medical condition, ailment, or disease. We must emphasize that any form of bodily introduction of these products into humans or animals is strictly prohibited by law. It is essential to adhere to these guidelines to ensure compliance with legal and ethical standards in research and experimentation.