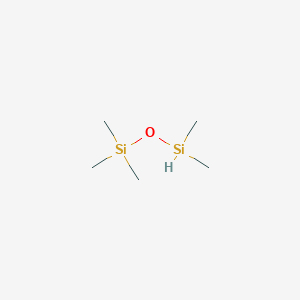
Pentamethyldisiloxane
Overview
Description
Pentamethyldisiloxane (PMDS, CAS 1438-82-0) is a linear organosiloxane with the formula (CH₃)₃Si-O-Si(CH₃)₂H. It is a colorless, flammable liquid with a boiling point of 84–86°C and a density of 0.758 g/cm³ . Its structure features a disiloxane backbone (Si-O-Si) with methyl substituents, rendering it highly hydrophobic and soluble in non-polar solvents like hexane . PMDS is widely used in hydrosilylation reactions, polymer synthesis, and as a precursor for functionalized siloxanes due to its reactive Si-H bond .
Preparation Methods
Grignard Reagent Method
Reaction Mechanism and Process Overview
The Grignard reagent method, detailed in CN101875662A, involves a two-step synthesis:
-
Grignard Reaction : High-hydrogen silicone oil (structural formula III) reacts with methylmagnesium halide (RMgX) in an ether solvent (e.g., tetrahydrofuran) with a cosolvent (e.g., toluene).
-
Hydrolysis : The intermediate product undergoes hydrolysis in acidic conditions to yield pentamethyldisiloxane .
The reaction proceeds as follows:
Optimization of Reaction Conditions
Key parameters influencing yield and purity include:
In a representative example, reacting 0.3 moles of magnesium with 0.47 moles of methyl bromide in toluene/ether (1:1) at 20°C yielded 7 g of this compound (50% yield) with >98% purity after distillation .
Advantages and Limitations
Advantages :
-
Utilizes cost-effective high-hydrogen silicone oil instead of expensive chlorosilanes .
-
Achieves higher yields (50%) compared to traditional hydrolysis methods (<20%) .
Limitations : -
Requires stringent moisture control for Grignard reagent stability.
-
Generates magnesium byproducts, necessitating post-reaction neutralization .
Acid-Catalyzed Rearrangement Method
Reaction Design and Catalytic System
CN107474067A describes a solvent-free method using 1,1,3,3-tetramethyldisiloxane (TMDS) and hexamethyldisiloxane (HMDO) under acidic conditions:
Concentrated sulfuric acid (10–40°C) facilitates the rearrangement, with a typical reactant ratio of 1:1–1:2 (TMDS:HMDO) .
Process Optimization and Yield
Critical factors include:
In Example 1, reacting 134 g TMDS and 162 g HMDO with 23.68 g H₂SO₄ at 10°C for 8 hours produced 50.7% this compound in the crude mixture, refining to 99% purity after distillation .
Advantages and Limitations
Advantages :
-
Environmentally favorable with minimal waste .
Limitations : -
Requires precise distillation to separate similarly boiling byproducts (e.g., HMDO) .
Comparative Analysis of Methods
Yield and Purity
Method | Yield (%) | Purity (%) | Key Byproducts |
---|---|---|---|
Grignard Reagent | 50 | >98 | Mg salts, siloxanes |
Acid-Catalyzed | 35–38 | >99 | HMDO, TMDS |
Economic and Industrial Considerations
-
Raw Material Costs : The Grignard method uses silicone oil (~$50/kg) and methylmagnesium bromide (~$200/kg), while the acid method employs TMDS (~$100/kg) and HMDO (~$80/kg) .
-
Scalability : The acid method’s simpler setup favors large-scale production despite lower yields .
-
Environmental Impact : The acid method generates less hazardous waste, aligning with green chemistry principles .
Industrial-Scale Production Recommendations
For high-volume output, the acid-catalyzed method is preferable due to its operational simplicity and lower capital costs. However, the Grignard method remains viable for specialty applications requiring higher yields. Future research should explore hybrid catalysts or continuous-flow systems to enhance efficiency.
Chemical Reactions Analysis
Piers–Rubinsztajn Reaction
The Piers–Rubinsztajn reaction is a cornerstone of PMDSO’s utility, enabling the cleavage of methyl ethers (e.g., methoxy groups) under mild conditions. This reaction involves PMDSO and a boron-based catalyst (e.g., B(C₆F₅)₃) to replace methyl groups with siloxy moieties, followed by acidic hydrolysis to regenerate hydroxyl groups .
Key Features:
-
Mechanism : PMDSO reacts with methyl ethers via nucleophilic attack, forming silyl ethers that are subsequently hydrolyzed.
-
Advantages :
-
Example : In the synthesis of 7-hydroxy-cannabidiol, PMDSO facilitated the removal of methoxy groups while maintaining the integrity of allylic alcohols .
Reaction Conditions and Yields:
Reaction Component | Details | Yield |
---|---|---|
Catalyst | B(C₆F₅)₃ or Cp*Si⁺·X⁻ (e.g., tris(pentafluorophenyl)borane) | - |
Solvent | Hexane or toluene | - |
Temperature | Room temperature to 60°C | - |
Product | Hydroxylated compounds (e.g., 7-hydroxy-cannabidiol) | Up to 31% |
Hydrosilylation and Cyclization
PMDSO participates in palladium-catalyzed hydrosilylation reactions, forming silylated carbocycles from functionalized dienes. These intermediates can be hydrolyzed to alcohols .
Reaction Workflow:
-
Hydrosilylation : PMDSO reacts with dienes (e.g., 1,3-dienes) in the presence of a palladium catalyst (e.g., (N–N)Pd(Me)Cl) to form silylated carbocycles.
-
Hydrolysis : Treatment with KF and peracetic acid converts silylated products into alcohols with retained stereochemistry.
Example Reaction:
Advantages:
-
High stereoselectivity in carbocycle formation.
-
Compatible with functionalized dienes (e.g., esters, amides) .
Silylation of Vinyl Arenes
PMDSO reacts with vinyl arenes in the presence of sodium triethylborohydride (NaHBEt₃) to form silylated products. This reaction proceeds via a carbanion intermediate, enabling efficient silylation .
Key Observations:
-
Regioselectivity : The reaction favors silylation at the less substituted silicon atom in PMDSO.
-
Catalyst Role : BEt₃ facilitates Si–O bond cleavage, enabling nucleophilic attack by carbanions.
Reaction Conditions:
Parameter | Details |
---|---|
Base | NaHBEt₃ (1:1 ratio with PMDSO) |
Solvent | THF or DMF |
Temperature | Room temperature |
Major Product | Dimethyl(1-phenylethyl)silane |
Oxidation and Substitution Reactions
PMDSO undergoes oxidation to form siloxane-based compounds and participates in substitution reactions .
Oxidation:
-
Reaction : PMDSO reacts with oxidizing agents (e.g., hydrogen peroxide) to form siloxane oxides.
-
Product : Siloxane-based derivatives with altered Si–O bonding.
Substitution:
-
Reaction : PMDSO undergoes substitution of methyl groups with other functional groups (e.g., halogens, organometallic reagents).
-
Reagents : Halogens (e.g., Cl₂), organometallic compounds (e.g., Grignard reagents).
Example:
PMDSO reacts with dimethylchlorosilane in the presence of potassium trimethylsilanolate to form high-purity siloxanes .
Hazardous Reactions and Stability
PMDSO exhibits reactivity under specific conditions:
Scientific Research Applications
Chemistry
PMDS plays a crucial role in the synthesis of siloxane-polyether copolymer surfactants and serves as a source of the trimethylsilyl functional group. Its unique reactivity allows for the formation of complex molecular structures essential for various chemical processes .
Biology
In biological research, PMDS is utilized in the development of biocompatible materials. It serves as a reagent in biochemical assays and is investigated for its potential use in drug delivery systems due to its ability to form stable bonds with biological molecules .
Medicine
The compound is being explored for its applications in medical devices and drug delivery systems. Its properties facilitate the encapsulation and release of therapeutic agents, enhancing the efficacy of treatments .
Industry
PMDS is extensively used in the production of silicone elastomers, lubricants, coatings, and personal care products. Its versatility allows for modifications that enhance performance characteristics tailored to specific applications .
Antimicrobial Properties
Recent studies have highlighted PMDS's antimicrobial potential. Derivatives of PMDS have shown significant antibacterial and antifungal activities. For instance:
- Triazole-PMDS Derivative : Exhibited antibacterial efficacy with zones of inhibition ranging from 15-20 mm against various bacterial strains.
- Imidazole-PMDS Derivative : Showed moderate antibacterial activity against Staphylococcus aureus and Escherichia coli.
Case Studies
- Synthesis of Triazole Derivatives : A study published in 2021 demonstrated that triazole derivatives synthesized from PMDS exhibited significant antibacterial properties against Gram-positive bacteria with minimum inhibitory concentrations (MICs) between 50 to 100 µg/mL.
- Alkylation Reactions : Research focused on alkylation reactions involving imidazole derivatives with PMDS revealed moderate antibacterial effects against common pathogens such as Staphylococcus aureus .
Toxicological Considerations
While PMDS shows promising biological activities, its safety profile is crucial for practical applications. Toxicological assessments indicate low acute toxicity; however, long-term exposure studies are necessary to fully understand its environmental impact and effects on human health .
Mechanism of Action
The mechanism of action of pentamethyldisiloxane involves its ability to form stable bonds with various functional groups. This property makes it an effective reagent in organic synthesis, where it can facilitate the formation of complex molecular structures. The compound’s molecular targets and pathways are primarily related to its interactions with other silicon-containing compounds and organic molecules .
Comparison with Similar Compounds
Structural and Physical Properties
Table 1: Key Properties of PMDS and Analogous Siloxanes
Key Differences :
- Reactivity : PMDS contains a reactive Si-H bond, enabling hydrosilylation and alkylation reactions, whereas hexamethyldisiloxane lacks this functionality .
- Volatility : PMDS has lower molecular weight and boiling point compared to trisiloxanes and pentasiloxanes, making it ideal for low-temperature syntheses .
- Solubility : PMDS’s compact structure enhances solubility in hexane, critical for polymer functionalization (e.g., regioregular polythiophenes) .
Table 2: Reaction Profiles of PMDS vs. Analogues
Key Findings :
Biological Activity
Pentamethyldisiloxane (PMDS) is a siloxane compound characterized by its unique structure and properties, which have garnered interest in various biological applications. This article explores the biological activity of PMDS, focusing on its antimicrobial properties, potential therapeutic applications, and relevant research findings.
Chemical Structure and Properties
This compound has the chemical formula and is represented by the structural formula:
This compound exhibits low viscosity and high thermal stability, making it suitable for various industrial applications, including as a lubricant and in personal care products.
Antimicrobial Activity
Recent studies have highlighted the antimicrobial potential of PMDS. Research indicates that PMDS derivatives exhibit significant antibacterial and antifungal activities. For instance, a study involving the reaction of PMDS with triazole derivatives demonstrated that the resulting compounds showed moderate to good antimicrobial activity against various strains of bacteria and fungi .
Table 1: Antimicrobial Activity of PMDS Derivatives
Compound | Activity Type | Efficacy (Zone of Inhibition) |
---|---|---|
Triazole-PMDS Derivative | Antibacterial | 15-20 mm |
Triazole-PMDS Derivative | Antifungal | 12-18 mm |
Imidazole-PMDS Derivative | Antibacterial | 10-15 mm |
The antimicrobial activity of PMDS is believed to be linked to its ability to disrupt cellular membranes and inhibit essential metabolic processes in microorganisms. The siloxane backbone may interact with lipid bilayers, leading to increased permeability and eventual cell lysis.
Case Studies
- Study on Triazole Derivatives : A study published in 2021 examined the synthesis of triazole derivatives from PMDS and their biological activity. The results indicated that these compounds possessed significant antibacterial properties against Gram-positive bacteria, with minimum inhibitory concentrations (MICs) ranging from 50 to 100 µg/mL .
- Alkylation Reactions : Another investigation focused on the alkylation of imidazole and benzimidazole derivatives with PMDS. The resulting organocyclosiloxane iodides were tested for their biological activity, revealing moderate antibacterial effects against Staphylococcus aureus and Escherichia coli .
Toxicological Considerations
While PMDS exhibits promising biological activities, it is essential to consider its safety profile. Toxicological assessments indicate that PMDS has low acute toxicity; however, long-term exposure studies are necessary to fully understand its effects on human health and the environment.
Q & A
Basic Research Questions
Q. What are the critical safety considerations when handling pentamethyldisiloxane in laboratory settings?
this compound is classified as a flammable liquid (UN 1993) with a flash point of 4.9°C . Researchers must store it in a cool, well-ventilated area away from ignition sources. Use inert gas purging during transfers to minimize combustion risks. Personal protective equipment (PPE), including flame-resistant lab coats and chemical-resistant gloves, is mandatory. Spill containment protocols should align with Class II hazardous material guidelines .
Q. How does the solubility profile of this compound influence its use in organic reactions?
this compound is insoluble in water but miscible with non-polar solvents like toluene and ethyl acetate . This property makes it suitable for hydrosilylation reactions in hydrophobic media. For example, in flow reactor syntheses, toluene is preferred as a solvent to ensure homogeneity and efficient mixing with alkenes .
Q. What analytical techniques are recommended for confirming the purity of this compound-derived products?
High-performance liquid chromatography (HPLC) and gas chromatography (GC) are standard for purity assessment. For example, post-synthesis purification of siloxane adducts involves vacuum distillation to remove excess this compound, followed by HPLC analysis to verify >95% purity . Nuclear magnetic resonance (NMR) spectroscopy is critical for structural confirmation, particularly for identifying brominated byproducts in degradation studies .
Advanced Research Questions
Q. How can steric hindrance in this compound derivatives impact reaction pathways, such as oxidative devulcanization?
Bulky substituents on silicon atoms (e.g., silicone T groups) hinder backside bromine attacks, leading to preferential formation of brominated this compound (compound 4) in degradation reactions. Comparative NMR analysis (3–4 ppm signals) and differential scanning calorimetry (DSC) can track steric effects on reaction kinetics . Mechanistic studies should contrast this compound with less hindered analogs (e.g., arylsilanes) to quantify steric contributions .
Q. What methodologies optimize this compound's role in Pt-catalyzed hydrosilylation for liquid crystal synthesis?
Key parameters include:
- Flow rate : 0.5 mL/min ensures optimal contact time in Pt@SiO₂ reactors .
- Solvent selection : Low-sulfur toluene minimizes catalyst poisoning .
- Post-reaction processing : Ethyl acetate rinsing prevents column clogging, while vacuum distillation (70°C, <5 mBar) isolates siloxane adducts efficiently . These steps yield mesomorphic siloxane-triphenylene hybrids with hexagonal columnar phases, confirmed via X-ray diffractometry .
Q. How does this compound enhance microsegregation in hybrid silicone-elastomer systems?
In hybrid materials (e.g., siloxane-triphenylene mesogens), this compound's methyl groups promote phase separation between hydrophobic siloxane domains and aromatic cores. This microsegregation stabilizes columnar mesophases (ColH—p6mm) up to 125°C, as demonstrated by DSC and polarized-light microscopy . Comparative studies with non-siloxane analogs show narrower mesophase ranges, highlighting this compound's structural advantage .
Q. What strategies mitigate side reactions during this compound-mediated rubber reduction?
In elastomer reduction, pre-swelling cryogenically ground rubber in dry toluene (30 min) improves siloxane penetration. Catalyst stock solutions (e.g., Pt-based) must be added post-swelling to minimize premature activation. Centrifugation (12,000 rpm, 20 min) and repeated toluene extraction remove undegraded polymer residues, ensuring >90% yield of soluble siloxane adducts .
Q. Data Contradiction and Resolution
Q. Discrepancies in reported reaction yields for this compound-based star oligomers: How can reproducibility be improved?
While yields of 94–97% are reported for star oligosiloxanes using tetrapropoxysilane , variations arise from inconsistent catalyst activation (e.g., moisture-sensitive B(C₆F₅)₃). Standardizing anhydrous conditions and quantifying residual moisture via Karl Fischer titration can resolve discrepancies. Additionally, monitoring reaction progress with real-time FTIR for Si-O-Si bond formation ensures endpoint accuracy .
Q. Why do competing pathways emerge in this compound-acrolein acetal reactions, and how are they controlled?
Competing 3,3-dimethoxypropyl vs. 2,2-dimethoxy-1-methylethyl adducts arise from Pt-catalyst regioselectivity. Selectivity is tuned by adjusting reaction temperature (20–60°C) and acetal stoichiometry. Hydrolysis of the former adduct yields siloxanylpropanals, while the latter undergoes Si-C cleavage to propionaldehyde. GC-MS analysis of volatile byproducts helps optimize conditions for desired pathways .
Q. Methodological Best Practices
Q. How should researchers document this compound-related syntheses to ensure reproducibility?
Follow the Beilstein Journal of Organic Chemistry guidelines:
- Experimental detail : Specify catalyst loading (e.g., 0.5 mol% Pt), solvent ratios, and purification steps (e.g., rotary evaporation parameters) .
- Data reporting : Include NMR shifts (¹H/¹³C), DSC thermograms, and chromatographic retention times. For novel compounds, provide elemental analysis or high-resolution mass spectrometry (HRMS) data .
- Safety protocols : Reference UN transport codes (e.g., Class 3 flammable liquid) and PPE requirements in supplementary materials .
Properties
IUPAC Name |
dimethylsilyloxy(trimethyl)silane | |
---|---|---|
Details | Computed by LexiChem 2.6.6 (PubChem release 2019.06.18) | |
Source | PubChem | |
URL | https://pubchem.ncbi.nlm.nih.gov | |
Description | Data deposited in or computed by PubChem | |
InChI |
InChI=1S/C5H16OSi2/c1-7(2)6-8(3,4)5/h7H,1-5H3 | |
Details | Computed by InChI 1.0.5 (PubChem release 2019.06.18) | |
Source | PubChem | |
URL | https://pubchem.ncbi.nlm.nih.gov | |
Description | Data deposited in or computed by PubChem | |
InChI Key |
AOJHDNSYXUZCCE-UHFFFAOYSA-N | |
Details | Computed by InChI 1.0.5 (PubChem release 2019.06.18) | |
Source | PubChem | |
URL | https://pubchem.ncbi.nlm.nih.gov | |
Description | Data deposited in or computed by PubChem | |
Canonical SMILES |
C[SiH](C)O[Si](C)(C)C | |
Details | Computed by OEChem 2.1.5 (PubChem release 2019.06.18) | |
Source | PubChem | |
URL | https://pubchem.ncbi.nlm.nih.gov | |
Description | Data deposited in or computed by PubChem | |
Molecular Formula |
C5H16OSi2 | |
Details | Computed by PubChem 2.1 (PubChem release 2019.06.18) | |
Source | PubChem | |
URL | https://pubchem.ncbi.nlm.nih.gov | |
Description | Data deposited in or computed by PubChem | |
Molecular Weight |
148.35 g/mol | |
Details | Computed by PubChem 2.1 (PubChem release 2021.05.07) | |
Source | PubChem | |
URL | https://pubchem.ncbi.nlm.nih.gov | |
Description | Data deposited in or computed by PubChem | |
CAS No. |
1438-82-0 | |
Record name | Pentamethyldisiloxane | |
Source | CAS Common Chemistry | |
URL | https://commonchemistry.cas.org/detail?cas_rn=1438-82-0 | |
Description | CAS Common Chemistry is an open community resource for accessing chemical information. Nearly 500,000 chemical substances from CAS REGISTRY cover areas of community interest, including common and frequently regulated chemicals, and those relevant to high school and undergraduate chemistry classes. This chemical information, curated by our expert scientists, is provided in alignment with our mission as a division of the American Chemical Society. | |
Explanation | The data from CAS Common Chemistry is provided under a CC-BY-NC 4.0 license, unless otherwise stated. | |
Retrosynthesis Analysis
AI-Powered Synthesis Planning: Our tool employs the Template_relevance Pistachio, Template_relevance Bkms_metabolic, Template_relevance Pistachio_ringbreaker, Template_relevance Reaxys, Template_relevance Reaxys_biocatalysis model, leveraging a vast database of chemical reactions to predict feasible synthetic routes.
One-Step Synthesis Focus: Specifically designed for one-step synthesis, it provides concise and direct routes for your target compounds, streamlining the synthesis process.
Accurate Predictions: Utilizing the extensive PISTACHIO, BKMS_METABOLIC, PISTACHIO_RINGBREAKER, REAXYS, REAXYS_BIOCATALYSIS database, our tool offers high-accuracy predictions, reflecting the latest in chemical research and data.
Strategy Settings
Precursor scoring | Relevance Heuristic |
---|---|
Min. plausibility | 0.01 |
Model | Template_relevance |
Template Set | Pistachio/Bkms_metabolic/Pistachio_ringbreaker/Reaxys/Reaxys_biocatalysis |
Top-N result to add to graph | 6 |
Feasible Synthetic Routes
Disclaimer and Information on In-Vitro Research Products
Please be aware that all articles and product information presented on BenchChem are intended solely for informational purposes. The products available for purchase on BenchChem are specifically designed for in-vitro studies, which are conducted outside of living organisms. In-vitro studies, derived from the Latin term "in glass," involve experiments performed in controlled laboratory settings using cells or tissues. It is important to note that these products are not categorized as medicines or drugs, and they have not received approval from the FDA for the prevention, treatment, or cure of any medical condition, ailment, or disease. We must emphasize that any form of bodily introduction of these products into humans or animals is strictly prohibited by law. It is essential to adhere to these guidelines to ensure compliance with legal and ethical standards in research and experimentation.