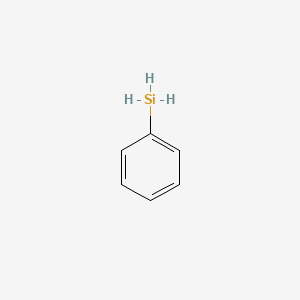
Phenylsilane
Overview
Description
Phenylsilane (PhSiH₃) is an organosilicon compound characterized by a silicon atom bonded to three hydrogen atoms and one phenyl group. Its Si–H bonds confer significant reactivity, making it a versatile reductant and precursor in organic synthesis, catalysis, and materials science. Key applications include:
- Catalytic Reductions: this compound serves as a terminal reductant in cobalt-catalyzed hydrosilylation of CO₂ to form silyl formates and methanol intermediates .
- Desulfinylation: In KOH-catalyzed systems, it selectively removes sulfinyl groups from α-sulfinylcarbonyl compounds with full regio- and stereoselectivity .
- Cross-Coupling Reactions: It participates in palladium-catalyzed couplings with aryl iodides to synthesize tris(aryl)phenylsilanes .
This compound’s low molecular weight and three Si–H equivalents per molecule enhance its efficiency in multi-step reductions, such as reductive C–N coupling of nitroaromatics .
Preparation Methods
Phenylsilane is typically synthesized in two steps from tetraethoxysilane (Si(OEt)₄). In the first step, phenylmagnesium bromide is added to form phenyltriethoxysilane (PhSi(OEt)₃) via a Grignard reaction. The resulting product is then reduced with lithium aluminum hydride (LiAlH₄) to afford this compound .
Synthetic Route:
- PhMgBr + Si(OEt)₄ → PhSi(OEt)₃ + MgBr(OEt)₄
- PhSi(OEt)₃ + 3 LiAlH₄ → PhSiH₃ + 3 LiAl(OEt)₄
Chemical Reactions Analysis
Reduction Reactions
Phenylsilane serves as a mild yet effective reductant in organic transformations, particularly for carbonyl groups and heteroatom-containing substrates.
Phosphine Oxide Reduction
This compound reduces tertiary phosphine oxides to tertiary phosphines with retention of configuration at phosphorus, making it valuable for synthesizing chiral phosphines :
Key Features :
-
Operates under mild conditions (room temperature, no aggressive reagents).
-
High functional group tolerance (e.g., esters, amines remain intact) .
Carbonyl Group Reduction
In the presence of transition-metal catalysts (e.g., Cu, Mn), this compound reduces ketones and aldehydes to alcohols. For example:
-
Methyl aryl ketones are reduced to secondary alcohols with 97% yield in propylene carbonate .
-
α,β-Unsaturated carbonyl compounds undergo selective 1,2-reduction to allylic alcohols using a Cu(I)-abnormal NHC catalyst .
Table 1: Reduction Efficiency of this compound
Substrate | Catalyst | Solvent | Yield (%) | Selectivity | Source |
---|---|---|---|---|---|
Methyl aryl ketones | None | Propylene | 97 | High | |
Cyclopropylcarboxylates | KOH | THF | 95 | Regiospecific | |
α,β-Unsaturated ketones | Cu(I)-NHC | Toluene | 92 | 1,2-Selective |
Hydrosilylation Reactions
This compound participates in hydrosilylation, adding Si–H bonds across unsaturated systems like alkenes and alkynes.
Catalytic Hydrosilylation
-
α,β-Unsaturated carbonyls : Forms allyl alcohols via 1,4-addition .
-
Azide-Alkyne Cycloaddition : Copper catalysts enable "click" chemistry for triazole synthesis .
Mechanistic Insight :
Hydrosilylation proceeds through radical intermediates in Mn- or Fe-catalyzed systems. This compound’s electrophilic silicon center facilitates H-atom transfer (HAT), enabling efficient alkene hydrogenation .
Radical Hydrofunctionalization
This compound acts as a hydride donor in metal-catalyzed radical reactions, enabling:
-
HAT Hydrogenation : Converts alkenes to alkanes using Mn or Fe catalysts. For example, (−)-terpineol is hydrogenated with 2,000 turnovers at 0.05 mol% Mn loading .
-
Radical-Polar Crossover : Combines radical addition with ionic steps for complex cyclizations (e.g., emindole SB synthesis) .
Key Advantage :
Unlike traditional reductants, this compound minimizes side reactions (e.g., over-reduction) due to its controlled reactivity .
Desulfinylation
This compound selectively removes sulfinyl groups under basic conditions:
Applications :
Enzymatic Reductions
This compound is compatible with biocatalysts, enabling enantioselective reductions:
-
Heterocyclic ketones (e.g., acetylpyridine) are reduced to chiral alcohols with >99% ee using engineered enzymes .
-
Fluoromethyl ketones undergo asymmetric reduction for pharmaceutical intermediates .
Comparative Reactivity
This compound outperforms other silanes in specific contexts:
Table 2: Silane Performance in HAT Hydrogenation
Silane | Solvent | Reaction Rate (mmol/h) | Diastereoselectivity (d.r.) |
---|---|---|---|
PhSiH₃ (this compound) | Hexane | 0.5 | 5.6→6.7 |
Ph(i-PrO)SiH₂ | Hexane | 12.4 | 7.2→8.9 |
PhSiH₃ | i-PrOH | 0.2 | 7.0→10.1 |
Notable Trends :
-
Ph(i-PrO)SiH₂ (isopropoxy derivative) shows 25× faster kinetics than this compound due to enhanced Si electrophilicity .
-
Polar solvents like i-PrOH reduce rates but improve selectivity .
This compound’s versatility stems from its balanced nucleophilicity, mild reducing power, and compatibility with diverse reaction conditions. Ongoing research continues to expand its applications in asymmetric synthesis, materials science, and green chemistry .
Scientific Research Applications
Applications Overview
The following table summarizes the primary applications of phenylsilane across different domains:
Pharmaceutical Applications
This compound has been utilized effectively in the modification of drug molecules. A study demonstrated its role as a reducing agent in the desulfinylation of sulfinyl-substituted cyclopropylcarboxylates, showcasing regioselectivity and efficiency in converting these compounds into alcohols . This method highlights this compound's potential for synthesizing pharmaceutical intermediates with improved properties.
Materials Science Innovations
In materials science, this compound is crucial for developing advanced coatings. It enhances the adhesion properties of coatings applied to various substrates. For instance, its incorporation into silicone rubber formulations has improved thermal stability and resistance to aging, making it ideal for high-performance applications like LED encapsulants .
Organic Synthesis Advances
This compound's versatility as a reagent is exemplified in its use for hydrosilylation reactions. Research indicates that it can facilitate the conversion of α,β-unsaturated carbonyl compounds into allyl alcohols with high yields under mild conditions, demonstrating its utility in organic chemistry .
Mechanism of Action
Phenylsilane exerts its effects primarily through its ability to donate hydride ions (H⁻). This property makes it a valuable reducing agent in organic synthesis. The hydride donation mechanism involves the transfer of a hydride ion from this compound to the substrate, resulting in the formation of reduced products .
Comparison with Similar Compounds
Isopropoxy(phenyl)silane (Ph(i-PrO)SiH₂)
- Structural Difference : Replaces one Si–H bond with an isopropoxy group.
- Reactivity : Outperforms phenylsilane in iron-catalyzed conjugate additions, enabling room-temperature reactions with higher yields (e.g., 85% vs. 45% for this compound) due to ligand effects stabilizing reactive intermediates .
- Selectivity : In total syntheses (e.g., aplydactone), Ph(i-PrO)SiH₂ achieves superior diastereoselectivity and yield in vinyl bromide reductions compared to this compound .
Trichlorothis compound (PhSiCl₃)
- Structural Difference : Replaces all three Si–H bonds with chlorine atoms.
- Reactivity : Mixed with this compound (3:1 ratio), it generates PhSiHCl₂ in situ, which exhibits higher oxophilicity for phosphoryl reductions . Chlorine atoms increase electrophilicity, accelerating reactions like alkynylphosphine synthesis.
Dithis compound (Ph₂SiH₂)
- Structural Difference : Two phenyl groups and two Si–H bonds.
- Applications : Less efficient than this compound in C–N coupling due to fewer Si–H equivalents (two vs. three), requiring higher stoichiometry for comparable yields . However, its bulkiness may improve selectivity in sterically demanding reactions.
Poly(methylhydrosiloxane) (PMHS)
- Structural Difference : A polymeric silane with repeating –Si(H)(CH₃)–O– units.
- Reactivity : Less reactive than this compound but cost-effective for large-scale reductions. In life cycle assessments (LCAs), PMHS shows lower environmental impact per mole of product but requires longer reaction times .
Methylthis compound (MePhSiH₂)
- Structural Difference : One methyl and one phenyl group on silicon.
- Enantioselectivity : In enzymatic ketone reductions, this compound achieves higher yields (99% vs. 78%) than methylthis compound, though enantioselectivity remains identical (98% e.e.) . The phenyl group may enhance substrate binding in the enzyme active site.
Key Data Tables
Table 1: Reactivity Comparison in Representative Reactions
Table 2: Physical and Functional Properties
Biological Activity
Phenylsilane, a silane compound characterized by its phenyl group, has garnered attention for its diverse biological activities. This article explores its biological properties, mechanisms of action, and potential applications, supported by research findings and case studies.
Overview of this compound
This compound (C6H5SiH3) is a silane compound that serves as a versatile reagent in organic synthesis. Its unique structure allows it to participate in various chemical reactions, including reductions and desulfinylation processes. Recent studies have highlighted its potential beyond synthetic applications, indicating significant biological activity.
Mechanisms of Biological Activity
- Reductive Properties : this compound has been shown to act as a reducing agent in various chemical reactions. For instance, it effectively converts sulfinyl-substituted cyclopropylcarboxylates into corresponding alcohols through a desulfinylation process, demonstrating regioselectivity and stereoselectivity . This property suggests potential applications in medicinal chemistry for modifying biologically active compounds.
- Cytotoxicity : Research indicates that this compound derivatives exhibit cytotoxic effects on different cancer cell lines. For example, certain derivatives have shown moderate activity against the HL-60 leukemia cell line, suggesting that this compound may contribute to the development of anticancer agents .
- Antibacterial Activity : Some studies have reported the antibacterial properties of this compound derivatives against various bacterial strains, including Bacillus subtilis. This activity is particularly relevant in the context of increasing antibiotic resistance .
Case Study 1: Desulfinylation Reactions
A study demonstrated the effectiveness of this compound in desulfinylation reactions under mild conditions. The presence of sulfinyl groups significantly altered the reaction pathway, leading to selective formation of desulfinylated products . This method offers a new approach for synthesizing compounds with potential therapeutic applications.
Case Study 2: Cytotoxicity Against Cancer Cells
A series of this compound derivatives were synthesized and tested for their cytotoxic effects on HL-60 cells. The results indicated that certain modifications to the phenyl group enhanced cytotoxicity, paving the way for further exploration of these compounds as potential anticancer agents .
Data Table: Summary of Biological Activities
Q & A
Basic Research Questions
Q. What are the key methodological considerations for synthesizing phenylsilane in laboratory settings?
this compound synthesis requires precise control of reaction conditions (e.g., inert atmosphere, temperature) and reagent selection. A common approach involves the reduction of phenylchlorosilane using lithium aluminum hydride (LiAlH4) under anhydrous conditions . Critical steps include:
- Purification : Distillation under reduced pressure to isolate this compound from byproducts.
- Characterization : Use <sup>1</sup>H NMR to confirm Si–H bonds (δ ~4.0 ppm) and FTIR for Si–H stretching vibrations (2100–2200 cm<sup>-1</sup>) .
- Safety : Adhere to protocols for handling pyrophoric reagents (e.g., LiAlH4) and ensure proper ventilation to avoid inhalation hazards .
Q. How should researchers characterize this compound’s purity and structural integrity?
A multi-technique approach is essential:
Q. What safety protocols are critical when handling this compound in experimental workflows?
- Personal Protective Equipment (PPE) : Gloves (nitrile), lab coats, and safety goggles to prevent skin/eye contact .
- Ventilation : Use fume hoods to mitigate vapor exposure (P261 precaution) .
- Spill Management : Neutralize spills with inert adsorbents (e.g., sand) and avoid water contact to prevent hydrolysis .
Advanced Research Questions
Q. How does this compound participate in catalytic N-formylation reactions, and what mechanistic insights are unresolved?
this compound acts as a hydride donor in CO2-mediated N-formylation, forming silyl ether intermediates. Key unresolved questions include:
- Active Sites : Whether Lewis acid/base pairs in ionic liquid catalysts enhance hydride transfer efficiency .
- Side Reactions : Competing pathways leading to siloxane byproducts under varying pressures (0.1–0.5 MPa) .
Methodological recommendations: - Use <sup>31</sup>P NMR to track phosphine oxide reduction (e.g., signal shifts from 23 ppm to −5 ppm) .
- Monitor CO2 uptake via in situ FTIR to correlate reaction progress with intermediate stability .
Q. How can researchers resolve contradictions in surface area data after this compound treatment of porous materials?
Discrepancies often arise from silicon contamination or incomplete pore activation. For example, TEM data may show structural collapse (surface area dropping to 0.03 m<sup>2</sup>/g) due to residual silicon blocking pores . Strategies:
- Post-Synthesis Analysis : Combine X-ray photoelectron spectroscopy (XPS) and elemental mapping to localize silicon residues.
- Control Experiments : Compare untreated vs. This compound-treated samples under identical activation conditions (e.g., thermal desorption profiles) .
Q. What computational frameworks are suitable for modeling this compound’s reactivity in organometallic systems?
- Density Functional Theory (DFT) : Calculate Si–H bond dissociation energies to predict hydride transfer kinetics.
- Molecular Dynamics (MD) : Simulate solvent effects on this compound’s stability in tetrahydrofuran (THF) vs. dichloromethane .
- Validation : Cross-reference computational results with experimental <sup>29</sup>Si NMR shifts (±5 ppm accuracy) .
Q. How can researchers address inconsistencies in catalytic performance across this compound-mediated reactions?
Variability often stems from:
- Impurity Profiles : Trace moisture or oxygen degrading this compound (validate via Karl Fischer titration) .
- Substrate Specificity : Steric effects in bulky amines reducing formylation yields .
Recommendations: - Standardize reaction conditions (e.g., glovebox purity, solvent drying).
- Use design of experiments (DoE) to optimize temperature, pressure, and catalyst loading .
Q. Methodological Guidelines
- Data Reporting : Follow NIH guidelines for experimental replicability, including raw data in appendices and processed data in main texts .
- Ethical Compliance : Obtain institutional approval for hazardous reagent protocols .
- Literature Review : Use Google Scholar to prioritize high-impact studies via citation rankings .
Properties
IUPAC Name |
phenylsilane | |
---|---|---|
Details | Computed by Lexichem TK 2.7.0 (PubChem release 2021.05.07) | |
Source | PubChem | |
URL | https://pubchem.ncbi.nlm.nih.gov | |
Description | Data deposited in or computed by PubChem | |
InChI |
InChI=1S/C6H8Si/c7-6-4-2-1-3-5-6/h1-5H,7H3 | |
Details | Computed by InChI 1.0.6 (PubChem release 2021.05.07) | |
Source | PubChem | |
URL | https://pubchem.ncbi.nlm.nih.gov | |
Description | Data deposited in or computed by PubChem | |
InChI Key |
PARWUHTVGZSQPD-UHFFFAOYSA-N | |
Details | Computed by InChI 1.0.6 (PubChem release 2021.05.07) | |
Source | PubChem | |
URL | https://pubchem.ncbi.nlm.nih.gov | |
Description | Data deposited in or computed by PubChem | |
Canonical SMILES |
C1=CC=C(C=C1)[SiH3] | |
Details | Computed by OEChem 2.3.0 (PubChem release 2021.05.07) | |
Source | PubChem | |
URL | https://pubchem.ncbi.nlm.nih.gov | |
Description | Data deposited in or computed by PubChem | |
Molecular Formula |
C6H8Si | |
Details | Computed by PubChem 2.1 (PubChem release 2021.05.07) | |
Source | PubChem | |
URL | https://pubchem.ncbi.nlm.nih.gov | |
Description | Data deposited in or computed by PubChem | |
Related CAS |
80731-82-4 | |
Details | Compound: Phenylsilane homopolymer | |
Record name | Phenylsilane homopolymer | |
Source | CAS Common Chemistry | |
URL | https://commonchemistry.cas.org/detail?cas_rn=80731-82-4 | |
Description | CAS Common Chemistry is an open community resource for accessing chemical information. Nearly 500,000 chemical substances from CAS REGISTRY cover areas of community interest, including common and frequently regulated chemicals, and those relevant to high school and undergraduate chemistry classes. This chemical information, curated by our expert scientists, is provided in alignment with our mission as a division of the American Chemical Society. | |
Explanation | The data from CAS Common Chemistry is provided under a CC-BY-NC 4.0 license, unless otherwise stated. | |
Molecular Weight |
108.21 g/mol | |
Details | Computed by PubChem 2.1 (PubChem release 2021.05.07) | |
Source | PubChem | |
URL | https://pubchem.ncbi.nlm.nih.gov | |
Description | Data deposited in or computed by PubChem | |
CAS No. |
694-53-1 | |
Record name | Phenylsilane | |
Source | CAS Common Chemistry | |
URL | https://commonchemistry.cas.org/detail?cas_rn=694-53-1 | |
Description | CAS Common Chemistry is an open community resource for accessing chemical information. Nearly 500,000 chemical substances from CAS REGISTRY cover areas of community interest, including common and frequently regulated chemicals, and those relevant to high school and undergraduate chemistry classes. This chemical information, curated by our expert scientists, is provided in alignment with our mission as a division of the American Chemical Society. | |
Explanation | The data from CAS Common Chemistry is provided under a CC-BY-NC 4.0 license, unless otherwise stated. | |
Disclaimer and Information on In-Vitro Research Products
Please be aware that all articles and product information presented on BenchChem are intended solely for informational purposes. The products available for purchase on BenchChem are specifically designed for in-vitro studies, which are conducted outside of living organisms. In-vitro studies, derived from the Latin term "in glass," involve experiments performed in controlled laboratory settings using cells or tissues. It is important to note that these products are not categorized as medicines or drugs, and they have not received approval from the FDA for the prevention, treatment, or cure of any medical condition, ailment, or disease. We must emphasize that any form of bodily introduction of these products into humans or animals is strictly prohibited by law. It is essential to adhere to these guidelines to ensure compliance with legal and ethical standards in research and experimentation.