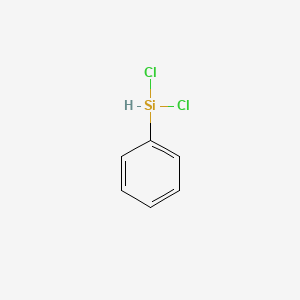
Dichloro(phenyl)silane
Overview
Description
Dichloro(phenyl)silane, also known as phenyl dichlorosilane, is an organosilicon compound with the chemical formula C6H5SiCl2. It is a colorless to pale yellow liquid that is used as an intermediate in the synthesis of various silicon-based materials. This compound is known for its reactivity due to the presence of silicon-chlorine bonds, making it a valuable reagent in organic and inorganic chemistry.
Preparation Methods
Synthetic Routes and Reaction Conditions
Dichloro(phenyl)silane can be synthesized through several methods. One common method involves the reaction of phenylmagnesium bromide with silicon tetrachloride. The reaction proceeds as follows:
C6H5MgBr+SiCl4→C6H5SiCl3+MgBrCl
The resulting trichlorophenylsilane can then be reduced using lithium aluminum hydride to yield this compound:
C6H5SiCl3+LiAlH4→C6H5SiCl2+LiAlHCl2
Industrial Production Methods
In industrial settings, this compound is typically produced by the direct reaction of phenylsilane with chlorine gas. This method is efficient and scalable, making it suitable for large-scale production:
C6H5SiH3+Cl2→C6H5SiCl2+HCl
Chemical Reactions Analysis
Types of Reactions
Dichloro(phenyl)silane undergoes various types of chemical reactions, including:
Hydrolysis: Reacts with water to form silanols and hydrochloric acid.
Reduction: Can be reduced to phenylsilane using reducing agents like lithium aluminum hydride.
Substitution: Reacts with nucleophiles to replace chlorine atoms with other functional groups.
Common Reagents and Conditions
Hydrolysis: Water or aqueous solutions.
Reduction: Lithium aluminum hydride in anhydrous ether.
Substitution: Nucleophiles such as amines or alcohols under mild conditions.
Major Products Formed
Hydrolysis: Phenylsilanediol and hydrochloric acid.
Reduction: Phenylsilane.
Substitution: Various organosilicon compounds depending on the nucleophile used.
Scientific Research Applications
Dichloro(phenyl)silane has a wide range of applications in scientific research and industry:
Chemistry: Used as a precursor for the synthesis of siloxanes and other organosilicon compounds.
Biology: Employed in the modification of biomolecules for enhanced stability and reactivity.
Medicine: Investigated for its potential use in drug delivery systems and as a component in medical devices.
Industry: Utilized in the production of silicone polymers, coatings, and adhesives.
Mechanism of Action
The reactivity of dichloro(phenyl)silane is primarily due to the presence of silicon-chlorine bonds. These bonds are highly polar, making the silicon atom electrophilic and susceptible to nucleophilic attack. The mechanism of action typically involves the nucleophilic substitution of chlorine atoms, leading to the formation of new silicon-based compounds.
Comparison with Similar Compounds
Similar Compounds
Phenylsilane: Similar structure but lacks chlorine atoms, making it less reactive.
Dichlorodiphenylsilane: Contains two phenyl groups, resulting in different reactivity and applications.
Trichlorophenylsilane: Contains an additional chlorine atom, making it more reactive and suitable for different synthetic applications.
Uniqueness
Dichloro(phenyl)silane is unique due to its balanced reactivity, making it versatile for various chemical transformations. Its ability to undergo hydrolysis, reduction, and substitution reactions makes it a valuable intermediate in both academic research and industrial applications.
Properties
IUPAC Name |
dichloro(phenyl)silane | |
---|---|---|
Details | Computed by Lexichem TK 2.7.0 (PubChem release 2021.05.07) | |
Source | PubChem | |
URL | https://pubchem.ncbi.nlm.nih.gov | |
Description | Data deposited in or computed by PubChem | |
InChI |
InChI=1S/C6H6Cl2Si/c7-9(8)6-4-2-1-3-5-6/h1-5,9H | |
Details | Computed by InChI 1.0.6 (PubChem release 2021.05.07) | |
Source | PubChem | |
URL | https://pubchem.ncbi.nlm.nih.gov | |
Description | Data deposited in or computed by PubChem | |
InChI Key |
VIRVTHOOZABTPR-UHFFFAOYSA-N | |
Details | Computed by InChI 1.0.6 (PubChem release 2021.05.07) | |
Source | PubChem | |
URL | https://pubchem.ncbi.nlm.nih.gov | |
Description | Data deposited in or computed by PubChem | |
Canonical SMILES |
C1=CC=C(C=C1)[SiH](Cl)Cl | |
Details | Computed by OEChem 2.3.0 (PubChem release 2021.05.07) | |
Source | PubChem | |
URL | https://pubchem.ncbi.nlm.nih.gov | |
Description | Data deposited in or computed by PubChem | |
Molecular Formula |
C6H6Cl2Si | |
Details | Computed by PubChem 2.1 (PubChem release 2021.05.07) | |
Source | PubChem | |
URL | https://pubchem.ncbi.nlm.nih.gov | |
Description | Data deposited in or computed by PubChem | |
Related CAS |
99936-07-9 | |
Details | Compound: Benzene, (dichlorosilyl)-, homopolymer | |
Record name | Benzene, (dichlorosilyl)-, homopolymer | |
Source | CAS Common Chemistry | |
URL | https://commonchemistry.cas.org/detail?cas_rn=99936-07-9 | |
Description | CAS Common Chemistry is an open community resource for accessing chemical information. Nearly 500,000 chemical substances from CAS REGISTRY cover areas of community interest, including common and frequently regulated chemicals, and those relevant to high school and undergraduate chemistry classes. This chemical information, curated by our expert scientists, is provided in alignment with our mission as a division of the American Chemical Society. | |
Explanation | The data from CAS Common Chemistry is provided under a CC-BY-NC 4.0 license, unless otherwise stated. | |
Molecular Weight |
177.10 g/mol | |
Details | Computed by PubChem 2.1 (PubChem release 2021.05.07) | |
Source | PubChem | |
URL | https://pubchem.ncbi.nlm.nih.gov | |
Description | Data deposited in or computed by PubChem | |
CAS No. |
1631-84-1 | |
Record name | (Dichlorosilyl)benzene | |
Source | CAS Common Chemistry | |
URL | https://commonchemistry.cas.org/detail?cas_rn=1631-84-1 | |
Description | CAS Common Chemistry is an open community resource for accessing chemical information. Nearly 500,000 chemical substances from CAS REGISTRY cover areas of community interest, including common and frequently regulated chemicals, and those relevant to high school and undergraduate chemistry classes. This chemical information, curated by our expert scientists, is provided in alignment with our mission as a division of the American Chemical Society. | |
Explanation | The data from CAS Common Chemistry is provided under a CC-BY-NC 4.0 license, unless otherwise stated. | |
Record name | Dichlorophenylsilane | |
Source | DTP/NCI | |
URL | https://dtp.cancer.gov/dtpstandard/servlet/dwindex?searchtype=NSC&outputformat=html&searchlist=139846 | |
Description | The NCI Development Therapeutics Program (DTP) provides services and resources to the academic and private-sector research communities worldwide to facilitate the discovery and development of new cancer therapeutic agents. | |
Explanation | Unless otherwise indicated, all text within NCI products is free of copyright and may be reused without our permission. Credit the National Cancer Institute as the source. | |
Retrosynthesis Analysis
AI-Powered Synthesis Planning: Our tool employs the Template_relevance Pistachio, Template_relevance Bkms_metabolic, Template_relevance Pistachio_ringbreaker, Template_relevance Reaxys, Template_relevance Reaxys_biocatalysis model, leveraging a vast database of chemical reactions to predict feasible synthetic routes.
One-Step Synthesis Focus: Specifically designed for one-step synthesis, it provides concise and direct routes for your target compounds, streamlining the synthesis process.
Accurate Predictions: Utilizing the extensive PISTACHIO, BKMS_METABOLIC, PISTACHIO_RINGBREAKER, REAXYS, REAXYS_BIOCATALYSIS database, our tool offers high-accuracy predictions, reflecting the latest in chemical research and data.
Strategy Settings
Precursor scoring | Relevance Heuristic |
---|---|
Min. plausibility | 0.01 |
Model | Template_relevance |
Template Set | Pistachio/Bkms_metabolic/Pistachio_ringbreaker/Reaxys/Reaxys_biocatalysis |
Top-N result to add to graph | 6 |
Feasible Synthetic Routes
Disclaimer and Information on In-Vitro Research Products
Please be aware that all articles and product information presented on BenchChem are intended solely for informational purposes. The products available for purchase on BenchChem are specifically designed for in-vitro studies, which are conducted outside of living organisms. In-vitro studies, derived from the Latin term "in glass," involve experiments performed in controlled laboratory settings using cells or tissues. It is important to note that these products are not categorized as medicines or drugs, and they have not received approval from the FDA for the prevention, treatment, or cure of any medical condition, ailment, or disease. We must emphasize that any form of bodily introduction of these products into humans or animals is strictly prohibited by law. It is essential to adhere to these guidelines to ensure compliance with legal and ethical standards in research and experimentation.