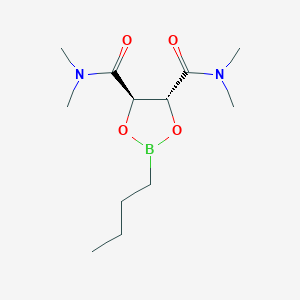
Butylboronic acid N,N,N',N'-tetramethyl-L-tartaric acid diamide ester
- Click on QUICK INQUIRY to receive a quote from our team of experts.
- With the quality product at a COMPETITIVE price, you can focus more on your research.
Overview
Description
Butylboronic acid N,N,N’,N’-tetramethyl-L-tartaric acid diamide ester is a boronic acid derivative with the empirical formula C12H23BN2O4 and a molecular weight of 270.13 g/mol . This compound is known for its application as a cyclopropanation ligand in organic synthesis, particularly in the total synthesis of natural products such as (+)-Frondosin and coibacins A and B .
Preparation Methods
Synthetic Routes and Reaction Conditions
The synthesis of butylboronic acid N,N,N’,N’-tetramethyl-L-tartaric acid diamide ester typically involves the reaction of butylboronic acid with N,N,N’,N’-tetramethyl-L-tartaric acid diamide under controlled conditions. The reaction is carried out in an organic solvent such as chloroform, and the product is purified through crystallization or chromatography .
Industrial Production Methods
Industrial production methods for this compound are not widely documented, but they likely involve similar synthetic routes with optimization for large-scale production. This includes the use of automated reactors and purification systems to ensure high yield and purity .
Chemical Reactions Analysis
Types of Reactions
Butylboronic acid N,N,N’,N’-tetramethyl-L-tartaric acid diamide ester undergoes various types of chemical reactions, including:
Cyclopropanation: It acts as a ligand in cyclopropanation reactions, facilitating the formation of cyclopropane rings.
Substitution Reactions: It can participate in substitution reactions where the boronic acid group is replaced by other functional groups.
Common Reagents and Conditions
Common reagents used in reactions involving this compound include:
Organometallic Reagents: Such as Grignard reagents and organolithium compounds.
Oxidizing Agents: For oxidation reactions, common oxidizing agents like hydrogen peroxide or m-chloroperbenzoic acid may be used.
Major Products
The major products formed from these reactions depend on the specific reaction conditions and reagents used. For example, cyclopropanation reactions yield cyclopropane derivatives, while substitution reactions can yield a variety of substituted boronic acid derivatives .
Scientific Research Applications
Butylboronic acid N,N,N’,N’-tetramethyl-L-tartaric acid diamide ester has several scientific research applications, including:
Organic Synthesis: It is used as a ligand in the synthesis of complex organic molecules, including natural products and pharmaceuticals.
Biological Research: It is used in the preparation of biologically active molecules for research purposes.
Pharmacological Studies: It is involved in the synthesis of pharmacologically active compounds, aiding in drug discovery and development.
Mechanism of Action
The mechanism of action of butylboronic acid N,N,N’,N’-tetramethyl-L-tartaric acid diamide ester involves its role as a ligand in catalytic reactions. It coordinates with metal catalysts to facilitate the formation of cyclopropane rings and other complex structures. The molecular targets and pathways involved include the activation of metal centers and the stabilization of reaction intermediates .
Comparison with Similar Compounds
Similar Compounds
Butylboronic Acid: A simpler boronic acid derivative used in organic synthesis.
N,N,N’,N’-Tetramethyl-L-tartaric Acid Diamide: A related compound used as a ligand in various catalytic reactions.
Uniqueness
Butylboronic acid N,N,N’,N’-tetramethyl-L-tartaric acid diamide ester is unique due to its specific combination of functional groups, which allows it to act as an effective ligand in cyclopropanation reactions. This makes it particularly valuable in the synthesis of complex natural products and pharmacologically active molecules .
Properties
IUPAC Name |
(4R,5R)-2-butyl-4-N,4-N,5-N,5-N-tetramethyl-1,3,2-dioxaborolane-4,5-dicarboxamide |
Source
|
---|---|---|
Details | Computed by LexiChem 2.6.6 (PubChem release 2019.06.18) | |
Source | PubChem | |
URL | https://pubchem.ncbi.nlm.nih.gov | |
Description | Data deposited in or computed by PubChem | |
InChI |
InChI=1S/C12H23BN2O4/c1-6-7-8-13-18-9(11(16)14(2)3)10(19-13)12(17)15(4)5/h9-10H,6-8H2,1-5H3/t9-,10-/m1/s1 |
Source
|
Details | Computed by InChI 1.0.5 (PubChem release 2019.06.18) | |
Source | PubChem | |
URL | https://pubchem.ncbi.nlm.nih.gov | |
Description | Data deposited in or computed by PubChem | |
InChI Key |
AFQWQRBBIZKYTE-NXEZZACHSA-N |
Source
|
Details | Computed by InChI 1.0.5 (PubChem release 2019.06.18) | |
Source | PubChem | |
URL | https://pubchem.ncbi.nlm.nih.gov | |
Description | Data deposited in or computed by PubChem | |
Canonical SMILES |
B1(OC(C(O1)C(=O)N(C)C)C(=O)N(C)C)CCCC |
Source
|
Details | Computed by OEChem 2.1.5 (PubChem release 2019.06.18) | |
Source | PubChem | |
URL | https://pubchem.ncbi.nlm.nih.gov | |
Description | Data deposited in or computed by PubChem | |
Isomeric SMILES |
B1(O[C@H]([C@@H](O1)C(=O)N(C)C)C(=O)N(C)C)CCCC |
Source
|
Details | Computed by OEChem 2.1.5 (PubChem release 2019.06.18) | |
Source | PubChem | |
URL | https://pubchem.ncbi.nlm.nih.gov | |
Description | Data deposited in or computed by PubChem | |
Molecular Formula |
C12H23BN2O4 |
Source
|
Details | Computed by PubChem 2.1 (PubChem release 2019.06.18) | |
Source | PubChem | |
URL | https://pubchem.ncbi.nlm.nih.gov | |
Description | Data deposited in or computed by PubChem | |
Molecular Weight |
270.14 g/mol |
Source
|
Details | Computed by PubChem 2.1 (PubChem release 2021.05.07) | |
Source | PubChem | |
URL | https://pubchem.ncbi.nlm.nih.gov | |
Description | Data deposited in or computed by PubChem | |
CAS No. |
161344-85-0 |
Source
|
Record name | (4R,5R)-2-Butyl-N,N,N',N'-tetramethyl-1,3,2-dioxaborolane-4,5-dicarboxamide | |
Source | European Chemicals Agency (ECHA) | |
URL | https://echa.europa.eu/information-on-chemicals | |
Description | The European Chemicals Agency (ECHA) is an agency of the European Union which is the driving force among regulatory authorities in implementing the EU's groundbreaking chemicals legislation for the benefit of human health and the environment as well as for innovation and competitiveness. | |
Explanation | Use of the information, documents and data from the ECHA website is subject to the terms and conditions of this Legal Notice, and subject to other binding limitations provided for under applicable law, the information, documents and data made available on the ECHA website may be reproduced, distributed and/or used, totally or in part, for non-commercial purposes provided that ECHA is acknowledged as the source: "Source: European Chemicals Agency, http://echa.europa.eu/". Such acknowledgement must be included in each copy of the material. ECHA permits and encourages organisations and individuals to create links to the ECHA website under the following cumulative conditions: Links can only be made to webpages that provide a link to the Legal Notice page. | |
Disclaimer and Information on In-Vitro Research Products
Please be aware that all articles and product information presented on BenchChem are intended solely for informational purposes. The products available for purchase on BenchChem are specifically designed for in-vitro studies, which are conducted outside of living organisms. In-vitro studies, derived from the Latin term "in glass," involve experiments performed in controlled laboratory settings using cells or tissues. It is important to note that these products are not categorized as medicines or drugs, and they have not received approval from the FDA for the prevention, treatment, or cure of any medical condition, ailment, or disease. We must emphasize that any form of bodily introduction of these products into humans or animals is strictly prohibited by law. It is essential to adhere to these guidelines to ensure compliance with legal and ethical standards in research and experimentation.