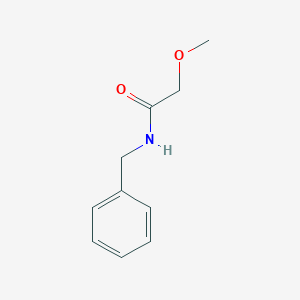
N-Benzyl-2-methoxyacetamide
Overview
Description
N-Benzyl-2-methoxyacetamide is an organic compound with the molecular formula C10H13NO2. It is an amide derivative that has been studied for its potential applications in various fields, including chemistry, biology, and industry. The compound is characterized by the presence of a benzyl group attached to the nitrogen atom and a methoxy group attached to the acetamide moiety.
Preparation Methods
Synthetic Routes and Reaction Conditions: N-Benzyl-2-methoxyacetamide can be synthesized through a one-pot biocatalytic amine transaminase/acyl transferase cascade. This method involves the formation of amides from the corresponding aldehydes or ketones in aqueous solution. The process utilizes enzymes such as acyl transferase from Mycobacterium smegmatis, which can perform trans-acylations in aqueous solution . The reaction conditions typically involve mild temperatures and the use of inexpensive starting materials, making it a cost-effective and environmentally friendly method.
Industrial Production Methods: The industrial production of this compound follows similar synthetic routes as described above. The use of biocatalytic methods is preferred due to their efficiency and sustainability. The process can achieve high conversions, up to 97%, making it suitable for large-scale production .
Chemical Reactions Analysis
Types of Reactions: N-Benzyl-2-methoxyacetamide undergoes various chemical reactions, including:
Oxidation: The compound can be oxidized to form corresponding carboxylic acids.
Reduction: Reduction reactions can convert the amide group to an amine group.
Substitution: The methoxy group can be substituted with other functional groups under appropriate conditions.
Common Reagents and Conditions:
Oxidation: Common oxidizing agents include potassium permanganate (KMnO4) and chromium trioxide (CrO3).
Reduction: Reducing agents such as lithium aluminum hydride (LiAlH4) and sodium borohydride (NaBH4) are commonly used.
Substitution: Substitution reactions often require catalysts and specific reaction conditions depending on the desired product.
Major Products Formed:
Oxidation: Formation of carboxylic acids.
Reduction: Formation of primary amines.
Substitution: Formation of various substituted derivatives depending on the substituent introduced.
Scientific Research Applications
N-Benzyl-2-methoxyacetamide has been explored for its applications in several scientific fields:
Chemistry: It is used as an intermediate in the synthesis of other organic compounds and as a reagent in various chemical reactions.
Medicine: Research is ongoing to explore its potential as a pharmaceutical intermediate and its role in drug development.
Mechanism of Action
The mechanism of action of N-Benzyl-2-methoxyacetamide involves its interaction with specific molecular targets and pathways. The compound can act as a substrate for enzymes such as acyl transferases and amine transaminases, facilitating the formation of amide bonds. The molecular targets include the active sites of these enzymes, where the compound undergoes catalytic transformations .
Comparison with Similar Compounds
N-Benzylacetamide: Similar structure but lacks the methoxy group.
N-Methoxyacetamide: Similar structure but lacks the benzyl group.
N-Benzyl-2-methoxyethanamide: Similar structure with an ethanamide moiety instead of acetamide.
Uniqueness: N-Benzyl-2-methoxyacetamide is unique due to the presence of both benzyl and methoxy groups, which confer specific reactivity and stability. This combination of functional groups makes it a versatile compound for various applications in research and industry.
Properties
IUPAC Name |
N-benzyl-2-methoxyacetamide | |
---|---|---|
Details | Computed by LexiChem 2.6.6 (PubChem release 2019.06.18) | |
Source | PubChem | |
URL | https://pubchem.ncbi.nlm.nih.gov | |
Description | Data deposited in or computed by PubChem | |
InChI |
InChI=1S/C10H13NO2/c1-13-8-10(12)11-7-9-5-3-2-4-6-9/h2-6H,7-8H2,1H3,(H,11,12) | |
Details | Computed by InChI 1.0.5 (PubChem release 2019.06.18) | |
Source | PubChem | |
URL | https://pubchem.ncbi.nlm.nih.gov | |
Description | Data deposited in or computed by PubChem | |
InChI Key |
VLAPGEODGZEQPC-UHFFFAOYSA-N | |
Details | Computed by InChI 1.0.5 (PubChem release 2019.06.18) | |
Source | PubChem | |
URL | https://pubchem.ncbi.nlm.nih.gov | |
Description | Data deposited in or computed by PubChem | |
Canonical SMILES |
COCC(=O)NCC1=CC=CC=C1 | |
Details | Computed by OEChem 2.1.5 (PubChem release 2019.06.18) | |
Source | PubChem | |
URL | https://pubchem.ncbi.nlm.nih.gov | |
Description | Data deposited in or computed by PubChem | |
Molecular Formula |
C10H13NO2 | |
Details | Computed by PubChem 2.1 (PubChem release 2019.06.18) | |
Source | PubChem | |
URL | https://pubchem.ncbi.nlm.nih.gov | |
Description | Data deposited in or computed by PubChem | |
Molecular Weight |
179.22 g/mol | |
Details | Computed by PubChem 2.1 (PubChem release 2021.05.07) | |
Source | PubChem | |
URL | https://pubchem.ncbi.nlm.nih.gov | |
Description | Data deposited in or computed by PubChem | |
Retrosynthesis Analysis
AI-Powered Synthesis Planning: Our tool employs the Template_relevance Pistachio, Template_relevance Bkms_metabolic, Template_relevance Pistachio_ringbreaker, Template_relevance Reaxys, Template_relevance Reaxys_biocatalysis model, leveraging a vast database of chemical reactions to predict feasible synthetic routes.
One-Step Synthesis Focus: Specifically designed for one-step synthesis, it provides concise and direct routes for your target compounds, streamlining the synthesis process.
Accurate Predictions: Utilizing the extensive PISTACHIO, BKMS_METABOLIC, PISTACHIO_RINGBREAKER, REAXYS, REAXYS_BIOCATALYSIS database, our tool offers high-accuracy predictions, reflecting the latest in chemical research and data.
Strategy Settings
Precursor scoring | Relevance Heuristic |
---|---|
Min. plausibility | 0.01 |
Model | Template_relevance |
Template Set | Pistachio/Bkms_metabolic/Pistachio_ringbreaker/Reaxys/Reaxys_biocatalysis |
Top-N result to add to graph | 6 |
Feasible Synthetic Routes
Disclaimer and Information on In-Vitro Research Products
Please be aware that all articles and product information presented on BenchChem are intended solely for informational purposes. The products available for purchase on BenchChem are specifically designed for in-vitro studies, which are conducted outside of living organisms. In-vitro studies, derived from the Latin term "in glass," involve experiments performed in controlled laboratory settings using cells or tissues. It is important to note that these products are not categorized as medicines or drugs, and they have not received approval from the FDA for the prevention, treatment, or cure of any medical condition, ailment, or disease. We must emphasize that any form of bodily introduction of these products into humans or animals is strictly prohibited by law. It is essential to adhere to these guidelines to ensure compliance with legal and ethical standards in research and experimentation.