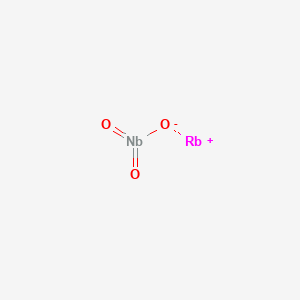
Rubidium niobate
Overview
Description
Rubidium niobate is a compound with the chemical formula RbNbO₃. It is known for its perovskite-type structure, which is a high-pressure phase of the compound.
Preparation Methods
Synthetic Routes and Reaction Conditions: Rubidium niobate can be synthesized through high-pressure and high-temperature methods. One common approach involves the synthesis of perovskite-type this compound at 1173 K and 4 GPa from non-perovskite this compound. This method involves single-crystal X-ray diffraction analysis to reveal the orthorhombic cell structure in the perovskite-type compound .
Industrial Production Methods: Industrial production of this compound typically involves the calcination of a reaction mixture containing rubidium and niobium precursors. The reaction mixture is heated to high temperatures, often around 1000°C, to achieve the desired phase of this compound .
Chemical Reactions Analysis
Types of Reactions: Rubidium niobate undergoes various types of chemical reactions, including oxidation, reduction, and substitution reactions. These reactions are influenced by the compound’s unique crystal structure and the presence of niobium in its composition.
Common Reagents and Conditions: Common reagents used in reactions involving this compound include oxygen, hydrogen, and various reducing agents. The reaction conditions often involve high temperatures and pressures to facilitate the desired chemical transformations .
Major Products Formed: The major products formed from reactions involving this compound depend on the specific reaction conditions and reagents used. For example, oxidation reactions may result in the formation of rubidium oxide and niobium oxide, while reduction reactions may yield rubidium metal and niobium metal .
Scientific Research Applications
Rubidium niobate has a wide range of scientific research applications due to its unique properties. Some of the key applications include:
Ferroelectric Materials: this compound is used in the design of ferroelectric materials, which have applications in memory devices, sensors, and actuators.
Nonlinear Optical Materials: The compound exhibits strong second harmonic generation signals, making it suitable for use in nonlinear optical materials and devices.
High-Pressure Research: this compound is studied under high-pressure conditions to understand its structural stability and phase transitions, which have implications for materials science and solid-state physics.
Electro-Optical Devices: Due to its high nonlinear optical and electro-optical properties, this compound is used in the development of electro-optical devices.
Mechanism of Action
The mechanism by which rubidium niobate exerts its effects is primarily related to its crystal structure and ferroelectric properties. The compound undergoes structural transitions from orthorhombic to tetragonal phases under varying temperature and pressure conditions. These transitions are associated with changes in the crystal lattice, which influence the compound’s ferroelectric and optical properties .
Molecular Targets and Pathways: The molecular targets and pathways involved in the mechanism of action of this compound include the alignment of dipoles within the crystal lattice and the generation of electric fields in response to external stimuli. These effects are crucial for the compound’s applications in ferroelectric and electro-optical devices .
Comparison with Similar Compounds
Potassium Niobate (KNbO₃): Similar ferroelectric properties but with different phase transition temperatures and crystal structures.
Barium Titanate (BaTiO₃): Known for its ferroelectric properties and used in similar applications, but with a different crystal structure and phase transition behavior.
Lead Vanadate (PbVO₃): Exhibits similar phase transitions under high-pressure conditions but has a different chemical composition and crystal structure.
Uniqueness of Rubidium Niobate: this compound is unique due to its high-pressure phase stability and the distinct structural transitions it undergoes. These properties make it a promising material for advanced ferroelectric and electro-optical applications .
Biological Activity
Rubidium niobate (RbNbO3) is a perovskite-type compound that has garnered attention due to its unique ferroelectric properties and potential applications in various fields, including electronics and optics. This article aims to provide a comprehensive overview of the biological activity of this compound, integrating data from recent studies, case analyses, and detailed research findings.
Overview of this compound
This compound is synthesized under high-pressure conditions, which significantly influence its structural and functional properties. The compound exhibits a complex crystal structure characterized by a non-centrosymmetric arrangement, crucial for its ferroelectric behavior. Recent advancements in synthesis techniques have enabled the production of RbNbO3 with enhanced dielectric properties, making it a candidate for various applications in electronics and optoelectronics.
Synthesis and Structural Characteristics
The synthesis of this compound typically involves the reaction of rubidium carbonate with niobium oxide at elevated temperatures and pressures. For instance, researchers have successfully synthesized RbNbO3 at temperatures around 1173 K (900°C) and pressures exceeding 40,000 atmospheres. The resulting material underwent a structural transformation from a triclinic phase to an orthorhombic perovskite structure, which is denser and exhibits improved dielectric polarization properties .
Table 1: Structural Parameters of this compound
Parameter | Value |
---|---|
Lattice constants (Å) | a = 3.9937(2) |
b = 5.8217(3) | |
c = 5.8647(2) | |
Density (g/cm³) | 5.514 |
Phase transitions | Orthorhombic to Tetragonal at 493 K |
The biological activity of this compound is primarily linked to its ionic constituents, particularly rubidium ions (Rb+), which can influence various physiological processes. Research has indicated that rubidium ions can interact with cellular mechanisms, affecting ion transport systems such as the Na+/K+ ATPase pump. Studies have shown that Rb+ can partially substitute for potassium ions in biological systems, potentially impacting cellular osmoregulation and excitability .
Case Studies
- Cellular Uptake Studies : In vitro experiments demonstrated that rubidium ions could enter rat red blood cells through specific ion channels. The influx of Rb+ was found to be influenced by external sodium concentrations and was sensitive to ouabain, indicating competitive interactions at the cellular membrane level .
- Toxicity Evaluations : Provisional toxicity assessments suggest that while rubidium compounds exhibit low toxicity at physiological concentrations, excessive exposure can lead to cellular disruption. The threshold for biological effects appears to be concentration-dependent, necessitating further investigation into safe exposure limits .
Research Findings
Recent studies have focused on the dielectric properties of this compound as they relate to its biological activity. The polarization characteristics observed in RbNbO3 are comparable to those of other ferroelectric materials like potassium niobate (KNbO3), suggesting potential applications in bioelectronic devices where ferroelectric materials could enhance signal transduction or energy harvesting from biological systems .
Table 2: Comparative Dielectric Properties
Material | Polarization (C/m²) | Dielectric Constant |
---|---|---|
This compound | 0.33 | Expected > KNbO3 |
Potassium Niobate | 0.32 | ~1000 |
Lithium Niobate | 0.71 | ~1000 |
Properties
IUPAC Name |
oxido(dioxo)niobium;rubidium(1+) | |
---|---|---|
Source | PubChem | |
URL | https://pubchem.ncbi.nlm.nih.gov | |
Description | Data deposited in or computed by PubChem | |
InChI |
InChI=1S/Nb.3O.Rb/q;;;-1;+1 | |
Source | PubChem | |
URL | https://pubchem.ncbi.nlm.nih.gov | |
Description | Data deposited in or computed by PubChem | |
InChI Key |
VSMYVMWMKWZYQD-UHFFFAOYSA-N | |
Source | PubChem | |
URL | https://pubchem.ncbi.nlm.nih.gov | |
Description | Data deposited in or computed by PubChem | |
Canonical SMILES |
[O-][Nb](=O)=O.[Rb+] | |
Source | PubChem | |
URL | https://pubchem.ncbi.nlm.nih.gov | |
Description | Data deposited in or computed by PubChem | |
Molecular Formula |
RbNbO3, NbO3Rb | |
Record name | rubidium niobate | |
Source | Wikipedia | |
URL | https://en.wikipedia.org/wiki/Dictionary_of_chemical_formulas | |
Description | Chemical information link to Wikipedia. | |
Source | PubChem | |
URL | https://pubchem.ncbi.nlm.nih.gov | |
Description | Data deposited in or computed by PubChem | |
Related CAS |
12031-63-9 (Parent) | |
Record name | Niobium rubidium oxide (NbRbO3) | |
Source | ChemIDplus | |
URL | https://pubchem.ncbi.nlm.nih.gov/substance/?source=chemidplus&sourceid=0012059517 | |
Description | ChemIDplus is a free, web search system that provides access to the structure and nomenclature authority files used for the identification of chemical substances cited in National Library of Medicine (NLM) databases, including the TOXNET system. | |
Molecular Weight |
226.372 g/mol | |
Source | PubChem | |
URL | https://pubchem.ncbi.nlm.nih.gov | |
Description | Data deposited in or computed by PubChem | |
CAS No. |
12059-51-7 | |
Record name | Niobium rubidium oxide (NbRbO3) | |
Source | ChemIDplus | |
URL | https://pubchem.ncbi.nlm.nih.gov/substance/?source=chemidplus&sourceid=0012059517 | |
Description | ChemIDplus is a free, web search system that provides access to the structure and nomenclature authority files used for the identification of chemical substances cited in National Library of Medicine (NLM) databases, including the TOXNET system. | |
Record name | Niobium rubidium oxide (NbRbO3) | |
Source | EPA Chemicals under the TSCA | |
URL | https://www.epa.gov/chemicals-under-tsca | |
Description | EPA Chemicals under the Toxic Substances Control Act (TSCA) collection contains information on chemicals and their regulations under TSCA, including non-confidential content from the TSCA Chemical Substance Inventory and Chemical Data Reporting. | |
Record name | Rubidium niobate | |
Source | European Chemicals Agency (ECHA) | |
URL | https://echa.europa.eu/substance-information/-/substanceinfo/100.031.838 | |
Description | The European Chemicals Agency (ECHA) is an agency of the European Union which is the driving force among regulatory authorities in implementing the EU's groundbreaking chemicals legislation for the benefit of human health and the environment as well as for innovation and competitiveness. | |
Explanation | Use of the information, documents and data from the ECHA website is subject to the terms and conditions of this Legal Notice, and subject to other binding limitations provided for under applicable law, the information, documents and data made available on the ECHA website may be reproduced, distributed and/or used, totally or in part, for non-commercial purposes provided that ECHA is acknowledged as the source: "Source: European Chemicals Agency, http://echa.europa.eu/". Such acknowledgement must be included in each copy of the material. ECHA permits and encourages organisations and individuals to create links to the ECHA website under the following cumulative conditions: Links can only be made to webpages that provide a link to the Legal Notice page. | |
Disclaimer and Information on In-Vitro Research Products
Please be aware that all articles and product information presented on BenchChem are intended solely for informational purposes. The products available for purchase on BenchChem are specifically designed for in-vitro studies, which are conducted outside of living organisms. In-vitro studies, derived from the Latin term "in glass," involve experiments performed in controlled laboratory settings using cells or tissues. It is important to note that these products are not categorized as medicines or drugs, and they have not received approval from the FDA for the prevention, treatment, or cure of any medical condition, ailment, or disease. We must emphasize that any form of bodily introduction of these products into humans or animals is strictly prohibited by law. It is essential to adhere to these guidelines to ensure compliance with legal and ethical standards in research and experimentation.