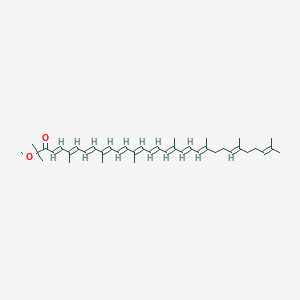
Spheroidenone
Overview
Description
Spheroidenone (C₄₁H₅₈O₂) is a keto-carotenoid predominantly found in photosynthetic bacteria such as Rhodobacter sphaeroides and members of the Roseobacter clade . It is synthesized under aerobic or high-light conditions through the oxidation of its precursor, spheroidene, via the introduction of a keto group at the C-2 position . This compound serves dual roles:
- Light harvesting: It transfers energy to bacteriochlorophyll (BChl) a in light-harvesting complexes (LHCs) with 92% efficiency .
- Photoprotection: It quenches singlet oxygen, mitigating oxidative damage under high-light or oxidative stress .
Its absorption spectrum spans 450–550 nm, with a bathochromic shift to 562 nm in the LH2 complex due to hydrogen bonding with protein residues .
Preparation Methods
Synthetic Routes and Reaction Conditions: Spheroidenone can be synthesized through the ketolation of spheroideneThe reaction typically requires specific catalysts and controlled conditions to ensure the correct placement of the keto group .
Industrial Production Methods: In an industrial setting, this compound production involves the cultivation of purple bacteria under semi-aerobic conditions. The bacteria naturally convert spheroidene to this compound when exposed to higher oxygen levels. This biotechnological approach leverages the bacteria’s metabolic pathways to produce this compound efficiently .
Chemical Reactions Analysis
Types of Reactions: Spheroidenone undergoes various chemical reactions, including:
Oxidation: this compound can be further oxidized to produce different derivatives.
Reduction: The keto group in this compound can be reduced to form spheroidene.
Substitution: Specific functional groups in this compound can be substituted with other groups under appropriate conditions.
Common Reagents and Conditions:
Oxidation: Common oxidizing agents include potassium permanganate and chromium trioxide.
Reduction: Reducing agents such as sodium borohydride or lithium aluminum hydride are used.
Substitution: Various reagents, depending on the desired substitution, can be employed.
Major Products:
Oxidation: Produces oxidized derivatives of this compound.
Reduction: Yields spheroidene.
Substitution: Results in substituted this compound derivatives.
Scientific Research Applications
Chemical Applications
Model Compound for Carotenoid Studies
Spheroidenone serves as a model compound in carotenoid chemistry and photochemistry. Its unique structure allows researchers to investigate the properties and behaviors of carotenoids under different conditions, contributing to a better understanding of these vital compounds in biological systems.
Photochemical Properties
Research indicates that this compound plays a crucial role in light-harvesting complexes of purple bacteria. It absorbs light energy and transfers it efficiently to bacteriochlorophyll, facilitating photosynthesis. The incorporation of this compound enhances energy transfer efficiency due to its strong interactions with protein residues in the light-harvesting complex .
Biological Applications
Role in Photosynthesis
this compound is integral to the photosynthetic process in Rhodobacter sphaeroides. When oxygen is present, spheroidene (its precursor) is converted to this compound, which alters the energy transfer pathways within the light-harvesting complexes. This conversion not only maintains the efficiency of photosynthesis but also provides photoprotection against oxidative damage .
Antioxidant Properties
In studies involving Rhodobacter sphaeroides, this compound has been shown to possess significant antioxidant properties. It interacts synergistically with other antioxidants, enhancing overall antioxidative activity while reducing cytotoxicity compared to other carotenoids like lycopene . This suggests potential applications in health supplements aimed at improving antioxidant defenses in humans.
Industrial Applications
Natural Pigment Production
this compound is utilized in the production of natural pigments for various industries, including food and cosmetics. Its vibrant color and stability under various conditions make it an attractive alternative to synthetic dyes.
Light-Harvesting Systems
The compound's ability to efficiently absorb and transfer light energy positions it as a valuable component in the development of advanced light-harvesting systems. These systems have potential applications in solar energy conversion technologies, where maximizing light absorption is critical.
Case Studies
Mechanism of Action
Spheroidenone exerts its effects through its role in the light-harvesting complexes of purple bacteria. It absorbs light and transfers the energy to bacteriochlorophyll, facilitating photosynthesis. The energy transfer efficiency is enhanced by the strong interactions between this compound and the protein residues in the light-harvesting complex .
Comparison with Similar Compounds
Structural and Functional Comparisons
Table 1: Key Features of Spheroidenone and Related Carotenoids
Mechanistic Insights
Energy Transfer Dynamics
- This compound transfers energy via the S₂ (1¹Bᵤ⁺) state to BChl a’s Qₓ state, accounting for 60% of total transfer . Its efficiency (92%) is lower than okenone (95%) and rhodopinal (~100%), likely due to differences in conjugation length and solvent-protein interactions .
- In contrast, spheroidene’s lower efficiency (~80–85%) stems from the absence of a keto group, which reduces polarizability and limits hydrogen bonding in LHCs .
Environmental Adaptation
- This compound replaces spheroidene under aerobic conditions, as its keto group enhances singlet oxygen quenching . This shift is critical for bacterial survival in high-light environments .
- Okenone and rhodopinal, prevalent in marine bacteria, exhibit higher efficiencies due to evolutionary optimization for low-light aquatic environments .
Spectral Properties
- This compound’s absorption spectrum splits into two forms in LH2: a "blue" form (522 nm) and a "red" form (562 nm). The latter arises from hydrogen bonding with residues like bArg29, increasing polarizability .
- Carotenoids with carbonyl groups (e.g., this compound, peridinin) exhibit broader absorption spectra and solvent-dependent excited-state dynamics compared to non-carbonyl analogs like spheroidene .
Research Implications and Gaps
- Industrial Applications: this compound’s antioxidant properties (e.g., in LycogenTM) make it valuable for nutraceuticals, though its yield in bacterial systems requires optimization .
- Unresolved Questions: The molecular basis for rhodopinal’s ~100% energy transfer efficiency remains unclear . The role of this compound’s "blue" spectral form in LH2 needs further study .
Biological Activity
Spheroidenone is a carotenoid pigment derived from spheroidene, primarily found in certain purple phototrophic bacteria such as Rhodobacter sphaeroides. This compound has garnered interest due to its unique structural properties and significant biological activities, particularly in the context of photosynthesis and photoprotection.
Chemical Structure and Properties
This compound features a complex structure characterized by multiple conjugated double bonds and a ketone group, which distinguishes it from other carotenoids. The presence of the carbonyl group (C=O) influences its spectral properties and interaction with light-harvesting proteins. The molecular formula of this compound is , and it has a notable absorption spectrum that allows it to participate effectively in light-harvesting processes.
Photochemical Behavior
This compound exhibits distinct photochemical characteristics that enhance its role in photosynthetic complexes. Research indicates that this compound can form complexes with light-harvesting proteins (LH2) in Rhodobacter sphaeroides, leading to high energy transfer efficiencies. Specifically, studies have shown that the carotenoid-to-bacteriochlorophyll (BChl) energy transfer efficiency in this compound-LH2 complexes is nearly 100%, making it highly effective in facilitating photosynthesis under varying environmental conditions .
Table 1: Energy Transfer Efficiency of Carotenoids in LH2 Complexes
Carotenoid | Number of Double Bonds (N) | Energy Transfer Efficiency (%) |
---|---|---|
Neurosporene | 9 | 94 |
Spheroidene | 10 | 96 |
This compound | 11 | 95 |
Lycopene | 11 | 64 |
Rhodopin | 11 | 62 |
Role in Photoprotection
One of the critical biological activities of this compound is its role as a photoprotective agent . It has been suggested that this compound can efficiently quench singlet oxygen, a reactive species generated during photosynthesis that can cause cellular damage. This capability is particularly important for organisms exposed to fluctuating light conditions, where excess light can lead to oxidative stress .
Case Study: Photoprotection Mechanism
In a study examining the photoprotective properties of carotenoids, this compound was found to effectively reduce singlet oxygen levels within the LH1 complex of Rhodobacter sphaeroides. The researchers demonstrated that while spheroidene provided some level of protection, this compound's enhanced quenching ability significantly improved the resilience of the bacterial cells against oxidative damage .
Biosynthesis and Metabolic Pathways
The biosynthesis of this compound involves several enzymatic steps starting from phytoene. In Rhodobacter sphaeroides, the enzyme phytoene desaturase catalyzes the conversion of phytoene into neurosporene, followed by further modifications leading to the production of spheroidene and ultimately this compound. Genetic studies have shown that manipulating these biosynthetic pathways can yield higher concentrations of this compound under specific growth conditions .
Table 2: Carotenoid Biosynthesis Pathway
Compound | Enzyme Involved | Number of Double Bonds (N) |
---|---|---|
Phytoene | Phytoene Desaturase | 3 |
Neurosporene | Phytoene Desaturase | 9 |
Spheroidene | Spheroidene Synthase | 10 |
This compound | Spheroidene Ketolase | 11 |
Q & A
Basic Research Questions
Q. What methodologies are used to quantify energy transfer efficiency from spheroidenone to bacteriochlorophyll (BChl) in light-harvesting complexes?
Energy transfer efficiency is measured via steady-state fluorescence excitation spectroscopy and absorptance (1-T) spectroscopy . By normalizing fluorescence excitation and absorptance spectra at the B850 band, the ratio of amplitudes across the carotenoid absorption range (450–550 nm) provides an efficiency value. For this compound in LH2 complexes, this yields ~92% efficiency due to optimized S₁→BChl energy transfer pathways .
Q. How does oxygen availability influence this compound biosynthesis in Rhodobacter sphaeroides?
this compound is synthesized under low-oxygen or oxidative stress conditions via enzymatic conversion of spheroidene by spheroidene monooxygenase. Its presence serves as a biomarker for microaerophilic environments in microbial ecology studies. This biosynthetic switch enhances photoprotection by enabling singlet oxygen quenching .
Q. What experimental techniques are employed to isolate and purify this compound-bound LH2 complexes?
LH2 complexes are isolated using ultrasonication to disrupt photosynthetic membranes, followed by differential centrifugation and ion-exchange chromatography . Purity is validated via absorption spectroscopy (e.g., Shimadzu UV-1800) and resonance Raman spectroscopy to confirm carotenoid-protein binding .
Advanced Research Questions
Q. How do spectral contradictions in this compound’s absorption profile in LH2 versus organic solvents inform its protein-environment interactions?
this compound exhibits a ~40 nm bathochromic shift in LH2 compared to solvents, attributed to hydrogen bonding with protein residues (e.g., Lys-Ile-Trp motifs) and high polarizability of the binding pocket. Transient absorption (TA) spectroscopy at cryogenic temperatures (77 K) reveals solvent-polarity-dependent shifts in excited-state absorption (ESA) bands, suggesting conformational stabilization of the keto group in s-trans configurations .
Q. What advanced kinetic models resolve contradictions in this compound’s excited-state lifetimes across LH complexes?
Target analysis of TA datasets applies microscopic decay rate constants to model this compound’s S₁(2¹A₉⁻) state dynamics. For LH2, lifetimes range from 800–900 fs, while LH1 shows biphasic decay (0.4 and 2.4 ps). These differences reflect variations in protein-induced conformational constraints and energy transfer pathways to BChl .
Q. How does conjugation length and keto-group incorporation affect this compound’s photoprotective role compared to non-keto carotenoids?
this compound’s extended conjugation (11 C=C bonds) and keto group lower the S₁ energy, enabling intramolecular charge transfer (ICT) states that enhance singlet oxygen quenching. Transient absorption spectroscopy in polar solvents (e.g., methanol) reveals ICT signatures (broad NIR features at 900–1200 nm), absent in spheroidene. This mechanism optimizes light-harvesting efficiency while mitigating oxidative damage .
Q. What experimental approaches differentiate “blue” and “red” this compound subpopulations in LH2 complexes?
Wavelength-selective TA spectroscopy at 520 nm (exciting “blue” form) and 565 nm (“red” form) isolates their species-associated difference spectra (SADS). “Blue” this compound (522 nm) arises from high polarizability, while “red” forms (562 nm) involve hydrogen-bond-stabilized ICT states. Spectral reconstruction using solvent-shifted reference spectra (e.g., 2-MTHF) validates these subpopulations .
Q. Methodological Considerations for Data Contradictions
Q. How can researchers address discrepancies in this compound’s S₂(1¹Bᵤ⁺) energy shifts across studies?
Discrepancies arise from solvent polarizability and protein-environment effects. Gaussian decomposition of absorption spectra and semiclassical quantum simulations (incorporating Huang-Rhys factors for vibrational modes) reconcile shifts by accounting for inhomogeneous broadening and pigment-protein interactions .
Q. Why do transient absorption studies report varying energy transfer efficiencies for this compound in LH1 versus LH2 complexes?
LH1’s tighter binding pocket stabilizes s-trans conformations, altering ICT state accessibility. Global fitting of TA datasets with multi-exponential decay models distinguishes contributions from competing pathways (e.g., S₁→BChl vs. S* states). LH2’s looser structure permits faster S₁ decay, enhancing energy transfer efficiency .
Properties
IUPAC Name |
(4E,6E,8E,10E,12E,14E,16E,18E,20E,22E,26E)-2-methoxy-2,6,10,14,19,23,27,31-octamethyldotriaconta-4,6,8,10,12,14,16,18,20,22,26,30-dodecaen-3-one | |
---|---|---|
Source | PubChem | |
URL | https://pubchem.ncbi.nlm.nih.gov | |
Description | Data deposited in or computed by PubChem | |
InChI |
InChI=1S/C41H58O2/c1-33(2)19-14-22-36(5)25-17-28-37(6)26-15-23-34(3)20-12-13-21-35(4)24-16-27-38(7)29-18-30-39(8)31-32-40(42)41(9,10)43-11/h12-13,15-16,18-21,23-27,29-32H,14,17,22,28H2,1-11H3/b13-12+,23-15+,24-16+,29-18+,32-31+,34-20+,35-21+,36-25+,37-26+,38-27+,39-30+ | |
Source | PubChem | |
URL | https://pubchem.ncbi.nlm.nih.gov | |
Description | Data deposited in or computed by PubChem | |
InChI Key |
ZQFURSYWJPLAJR-FZFXUSNISA-N | |
Source | PubChem | |
URL | https://pubchem.ncbi.nlm.nih.gov | |
Description | Data deposited in or computed by PubChem | |
Canonical SMILES |
CC(=CCCC(=CCCC(=CC=CC(=CC=CC=C(C)C=CC=C(C)C=CC=C(C)C=CC(=O)C(C)(C)OC)C)C)C)C | |
Source | PubChem | |
URL | https://pubchem.ncbi.nlm.nih.gov | |
Description | Data deposited in or computed by PubChem | |
Isomeric SMILES |
CC(=CCC/C(=C/CC/C(=C/C=C/C(=C/C=C/C=C(\C)/C=C/C=C(\C)/C=C/C=C(\C)/C=C/C(=O)C(C)(C)OC)/C)/C)/C)C | |
Source | PubChem | |
URL | https://pubchem.ncbi.nlm.nih.gov | |
Description | Data deposited in or computed by PubChem | |
Molecular Formula |
C41H58O2 | |
Source | PubChem | |
URL | https://pubchem.ncbi.nlm.nih.gov | |
Description | Data deposited in or computed by PubChem | |
Molecular Weight |
582.9 g/mol | |
Source | PubChem | |
URL | https://pubchem.ncbi.nlm.nih.gov | |
Description | Data deposited in or computed by PubChem | |
CAS No. |
13836-70-9 | |
Record name | Spheroidenone | |
Source | ChemIDplus | |
URL | https://pubchem.ncbi.nlm.nih.gov/substance/?source=chemidplus&sourceid=0013836709 | |
Description | ChemIDplus is a free, web search system that provides access to the structure and nomenclature authority files used for the identification of chemical substances cited in National Library of Medicine (NLM) databases, including the TOXNET system. | |
Retrosynthesis Analysis
AI-Powered Synthesis Planning: Our tool employs the Template_relevance Pistachio, Template_relevance Bkms_metabolic, Template_relevance Pistachio_ringbreaker, Template_relevance Reaxys, Template_relevance Reaxys_biocatalysis model, leveraging a vast database of chemical reactions to predict feasible synthetic routes.
One-Step Synthesis Focus: Specifically designed for one-step synthesis, it provides concise and direct routes for your target compounds, streamlining the synthesis process.
Accurate Predictions: Utilizing the extensive PISTACHIO, BKMS_METABOLIC, PISTACHIO_RINGBREAKER, REAXYS, REAXYS_BIOCATALYSIS database, our tool offers high-accuracy predictions, reflecting the latest in chemical research and data.
Strategy Settings
Precursor scoring | Relevance Heuristic |
---|---|
Min. plausibility | 0.01 |
Model | Template_relevance |
Template Set | Pistachio/Bkms_metabolic/Pistachio_ringbreaker/Reaxys/Reaxys_biocatalysis |
Top-N result to add to graph | 6 |
Feasible Synthetic Routes
Disclaimer and Information on In-Vitro Research Products
Please be aware that all articles and product information presented on BenchChem are intended solely for informational purposes. The products available for purchase on BenchChem are specifically designed for in-vitro studies, which are conducted outside of living organisms. In-vitro studies, derived from the Latin term "in glass," involve experiments performed in controlled laboratory settings using cells or tissues. It is important to note that these products are not categorized as medicines or drugs, and they have not received approval from the FDA for the prevention, treatment, or cure of any medical condition, ailment, or disease. We must emphasize that any form of bodily introduction of these products into humans or animals is strictly prohibited by law. It is essential to adhere to these guidelines to ensure compliance with legal and ethical standards in research and experimentation.