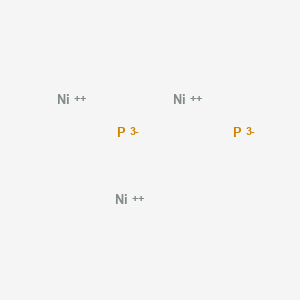
Dinickel phosphide
Overview
Description
Dinickel phosphide (Ni₂P) is a transition metal phosphide with a Pt-like electronic structure, making it a promising low-cost alternative to noble metal catalysts in hydrogenation and electrocatalytic reactions . Its hexagonal crystal structure exposes active Ni³⁺ and P sites, which facilitate hydrogen adsorption and charge transfer, critical for hydrogen evolution reactions (HER) and selective hydrogenation . Ni₂P nanoparticles synthesized via solvent-less routes or single-source precursors exhibit high stability in acidic media and retain catalytic activity over 1,000 cycles .
Preparation Methods
Synthetic Routes and Reaction Conditions: Dinickel phosphide can be synthesized through several methods, including thermal decomposition, solid-state reactions, and hydrothermal synthesis. One common method involves the thermal decomposition of nickel complexes in the presence of phosphorus sources. For example, single-crystalline this compound nanowires can be synthesized by the thermal decomposition of continuously delivered nickel-tri-octylphosphine complexes using a syringe pump .
Industrial Production Methods: In industrial settings, this compound is often produced through solid-state reactions. This involves mixing nickel acetate with red phosphorus and heating the mixture at high temperatures. Another method includes the use of nickel acetate and hexadecyl trimethyl ammonium bromide in a hydrothermal approach, resulting in the formation of this compound nanowires .
Chemical Reactions Analysis
Types of Reactions: Dinickel phosphide undergoes various chemical reactions, including oxidation, reduction, and substitution. It is particularly known for its catalytic activity in hydrogen evolution reactions and oxygen evolution reactions.
Common Reagents and Conditions:
Oxidation: this compound can be oxidized in the presence of oxygen or other oxidizing agents.
Reduction: It can be reduced using hydrogen or other reducing agents.
Substitution: Substitution reactions can occur with other metal phosphides or in the presence of suitable ligands.
Major Products Formed: The major products formed from these reactions depend on the specific conditions and reagents used. For example, in hydrogen evolution reactions, hydrogen gas is produced .
Scientific Research Applications
Electrocatalysis
Hydrogen Evolution Reaction (HER)
Ni₂P has emerged as a promising electrocatalyst for the hydrogen evolution reaction (HER), which is crucial for sustainable hydrogen production. Recent studies have demonstrated that Ni₂P exhibits excellent electrocatalytic activity due to its unique electronic structure and active sites.
- Performance Metrics : Research indicates that Ni₂P can achieve low overpotentials and high current densities, making it competitive with traditional noble metal catalysts. For instance, samarium-doped Ni₂P has shown enhanced HER performance with a lower onset potential and improved stability compared to pure Ni₂P .
- Mechanism Insights : The mechanism of HER on Ni₂P involves the adsorption of hydrogen ions on nickel sites, with density functional theory (DFT) calculations revealing that the nickel sites are primarily responsible for catalyzing the reaction .
Table 1: HER Performance of Ni₂P Variants
Catalyst | Onset Potential (mV) | Tafel Slope (mV/dec) | Stability (hours) |
---|---|---|---|
Pure Ni₂P | 94 | 113.2 | 50 |
Samarium-Doped Ni₂P | 85 | 100 | 100 |
Cu-Doped Ni₂P | 146 | 120 | 75 |
Heterogeneous Catalysis
Vapor Phase Reduction of Nitrobenzene
Ni₂P serves as an effective heterogeneous catalyst for the vapor phase reduction of nitrobenzene to aniline, a key intermediate in dye and pharmaceutical production. This reaction showcases the versatility of Ni₂P in catalyzing organic transformations.
- Reaction Conditions : The catalytic activity of Ni₂P in this process is attributed to its ability to facilitate hydrogenation reactions under mild conditions, providing a more sustainable alternative to traditional methods .
- Selectivity and Yield : Studies indicate that Ni₂P can achieve high selectivity for aniline with minimal by-products, highlighting its efficiency as a catalyst in organic synthesis.
Material Science
Nanostructuring and Composite Materials
The unique properties of Ni₂P allow it to be utilized in the development of advanced materials, particularly in creating nanostructured composites for energy storage applications.
- Supercapacitors : Research has shown that incorporating Ni₂P into composite materials enhances their electrochemical performance, making them suitable for supercapacitor applications due to their high specific capacitance and excellent cycling stability .
- Phase Transformations : The ability to induce phase transformations in Ni₂P through doping or varying synthesis conditions opens avenues for tailoring its properties for specific applications. For example, doping with transition metals like Cu or Mn can significantly alter its catalytic performance and stability .
Case Study 1: Samarium-Doped Ni₂P for HER
A recent study investigated the effects of samarium doping on the electrocatalytic properties of Ni₂P. The results demonstrated that samarium significantly improved the HER activity by modifying the electronic structure and enhancing charge transfer kinetics. This study emphasizes the importance of dopants in optimizing catalyst performance .
Case Study 2: Ni₂P in Organic Synthesis
In another study, Ni₂P was employed as a catalyst for the vapor phase reduction of nitrobenzene. The research highlighted its effectiveness in achieving high yields of aniline with reduced reaction times compared to conventional catalysts. This application underscores the potential of Ni₂P in industrial processes .
Mechanism of Action
The mechanism by which dinickel phosphide exerts its effects is primarily through its catalytic activity. In hydrogen evolution reactions, this compound acts as a catalyst, facilitating the reduction of protons to produce hydrogen gas. This process involves the adsorption of hydrogen ions onto the surface of the this compound, followed by electron transfer and the release of hydrogen gas .
Comparison with Similar Compounds
Comparison with Similar Nickel Phosphides
Catalytic Performance in Hydrogen Evolution Reactions (HER)
Ni₂P outperforms other nickel phosphides in HER due to its optimized d-band center and structural stability. Key comparisons include:
- Ni₂P vs. Ni₁₂P₅: Ni₂P requires 58% lower overpotential than Ni₁₂P₅ (174 vs. 450 mV) due to its hollow, faceted structure that exposes active (001) surfaces .
- Ni₂P vs. Ni₅P₄: While Ni₅P₄ shows marginally lower overpotentials in some studies (158 mV), Ni₂P exhibits superior stability (>1,000 cycles vs. 800 cycles) .
Selectivity and Activity in Hydrogenation Reactions
Ni₂P’s selectivity and activity are tunable via surface modifications. For example:
- Unmodified Ni₂P : Selectivity of 38.1% (3-nitrostyrene) and 21.3% (cinnamaldehyde) .
- SPhF-Chelated Ni₂P : Selectivity increases to ≈100% with 12× higher activity due to confined flat adsorption and downshifted d-band centers .
In contrast, Raney Ni (a commercial catalyst) achieves ≈100% selectivity only after SPhF chelation, but its activity remains half that of modified Ni₂P .
Key Factors Influencing Performance
Electronic and Steric Effects
- d-Band Center : Ni₂P’s downshifted d-band center weakens hydrogen adsorption, enhancing HER activity .
- Surface Modification : Chelation with SPhF arrays introduces steric effects, suppressing side reactions in hydrogenation .
Morphological Stability
Ni₂P’s hollow nanoparticles and epitaxial interfaces (e.g., with nickel thiophosphate nanosheets) reduce charge-transfer resistance and prevent aggregation during HER .
Biological Activity
Dinickel phosphide (Ni₂P) is a compound that has garnered attention in various fields, particularly in catalysis and materials science. Its unique properties and biological activities make it a subject of interest for researchers. This article explores the biological activity of Ni₂P, focusing on its applications, mechanisms, and relevant research findings.
Overview of this compound
This compound is a transition metal phosphide characterized by its unique structural and electronic properties. It has been extensively studied for its catalytic activity in various chemical reactions, including hydrogen evolution reactions (HER), alkylation processes, and as a potential agent in medicinal chemistry.
1. Catalytic Applications
Ni₂P has shown significant potential as a catalyst in biological and chemical reactions. Notably, it has been employed in the C-3 alkylation of oxindoles, which are compounds with potential applications as NMDA antagonists, indicating possible implications in neuropharmacology . The catalytic efficiency of Ni₂P can be enhanced through various modifications, such as doping with other elements like samarium, which improves its electrocatalytic performance for HER .
2. Electrocatalytic Properties
The electrocatalytic activity of Ni₂P is noteworthy. Studies have demonstrated that different phases of nickel phosphides exhibit varying catalytic efficiencies for HER. For example, Ni₅P₄ showed superior catalytic activity compared to Ni₂P and Ni₁₂P₅ due to its structural characteristics . The intrinsic activity and surface area of Ni₂P can be significantly improved through doping techniques, enhancing its suitability for applications in sustainable energy production .
3. Antitumor Activity
Research has indicated that phosphorus-based compounds, including nickel phosphides, may possess antitumor properties. A study highlighted the potential of metal phosphorus complexes as antitumor agents, suggesting avenues for further exploration in cancer treatment . The specific mechanisms through which Ni₂P exerts these effects are still under investigation but may involve interactions at the cellular level.
Research Findings
Table 1: Summary of Key Studies on this compound
Case Studies
-
C-3 Functionalized Oxindoles Synthesis :
A study utilized a cerium dioxide-supported Ni₂P catalyst to achieve a 95% yield in the synthesis of 3-benzyl-2-oxindole from benzyl alcohol at elevated temperatures . This demonstrates the compound's efficacy in organic synthesis with potential biological implications. -
Samarium-Doped Ni₂P :
Research indicated that samarium doping significantly improved the electrocatalytic performance of Ni₂P for HER, achieving notable efficiencies that could impact renewable energy technologies . This work underscores the importance of material modifications to enhance biological and chemical functionalities. -
Antitumor Properties :
Investigations into metal phosphorus complexes have revealed promising antitumor activities, suggesting that this compound may contribute to new therapeutic strategies against cancer .
Q & A
Basic Research Questions
Q. What are the common synthetic methods for preparing dinickel phosphide (Ni₂P) nanoparticles, and how do they influence crystallinity and morphology?
- Methodology :
- Solvothermal synthesis : Red phosphorus and nickel precursors are reacted in organic solvents (e.g., ethylene glycol) under high temperatures (180–220°C) with surfactants like cetyltrimethylammonium bromide (CTAB) to control particle size and dispersion .
- Mechanochemical synthesis : Ball-milling nickel chloride with sodium phosphide (Na₃P) under solvent-free conditions produces ultrasmall Ni₂P nanoparticles (3–5 nm) with high surface area .
- Phase-controlled synthesis : Adjusting the Ni/P precursor ratio and reaction time can yield different phases (e.g., Ni₂P vs. Ni₁₂P₅), which are verified via X-ray diffraction (XRD) and transmission electron microscopy (TEM) .
Q. Which characterization techniques are essential for validating Ni₂P synthesis and structural properties?
- Core techniques :
- XRD : Confirms phase purity and crystallinity by matching peak positions with reference patterns (e.g., JCPDS 89-2741 for Ni₂P) .
- TEM/HRTEM : Reveals nanoparticle size, morphology (e.g., hollow vs. solid structures), and lattice fringes (e.g., 0.22 nm spacing for Ni₂P(001)) .
- X-ray photoelectron spectroscopy (XPS) : Identifies surface oxidation states (e.g., Ni²⁺ vs. Ni⁰) and phosphorus bonding environments (Pδ⁻ in Ni₂P) .
Advanced Research Questions
Q. How can the hydrogen evolution reaction (HER) activity of Ni₂P be optimized through facet engineering and doping?
- Facet engineering : Ni₂P nanoparticles exposing the (001) facet exhibit lower HER overpotentials (e.g., 58 mV at 10 mA cm⁻²) due to optimal hydrogen adsorption energies, as predicted by DFT calculations .
- Transition-metal doping : Incorporating Mn or Co into Ni₂P alters electronic structure, reducing charge-transfer resistance. For example, Mn-doped Ni₂P achieves an overpotential of 158 mV, outperforming pristine Ni₂P (164 mV) .
- Methodological steps :
Synthesize doped Ni₂P via hot-injection or hydrothermal routes.
Validate doping efficiency via EDS and XPS.
Compare HER performance using linear sweep voltammetry (LSV) in 0.5 M H₂SO₄ .
Q. What experimental strategies address contradictions in reported HER overpotentials for Ni₂P-based catalysts?
- Root causes : Discrepancies arise from variations in nanoparticle size, exposed facets, electrolyte pH, and testing setups (e.g., reference electrode calibration) .
- Resolution strategies :
- Standardize HER testing protocols (e.g., IR compensation, scan rate).
- Report detailed synthesis parameters (precursor ratios, annealing time) to enable reproducibility .
Q. How is the long-term stability of Ni₂P electrocatalysts evaluated under acidic or alkaline conditions?
- Accelerated degradation tests :
- Cyclic voltammetry (CV) : 1,000 cycles in 0.5 M H₂SO₄ with <5% activity loss confirm stability .
- Chronoamperometry : Measure current density retention at fixed overpotentials for 24+ hours .
Q. Methodological Recommendations
Properties
IUPAC Name |
nickel(2+);phosphorus(3-) | |
---|---|---|
Source | PubChem | |
URL | https://pubchem.ncbi.nlm.nih.gov | |
Description | Data deposited in or computed by PubChem | |
InChI |
InChI=1S/3Ni.2P/q3*+2;2*-3 | |
Source | PubChem | |
URL | https://pubchem.ncbi.nlm.nih.gov | |
Description | Data deposited in or computed by PubChem | |
InChI Key |
JCZGSHVDNCPPKK-UHFFFAOYSA-N | |
Source | PubChem | |
URL | https://pubchem.ncbi.nlm.nih.gov | |
Description | Data deposited in or computed by PubChem | |
Canonical SMILES |
[P-3].[P-3].[Ni+2].[Ni+2].[Ni+2] | |
Source | PubChem | |
URL | https://pubchem.ncbi.nlm.nih.gov | |
Description | Data deposited in or computed by PubChem | |
Molecular Formula |
Ni3P2 | |
Source | PubChem | |
URL | https://pubchem.ncbi.nlm.nih.gov | |
Description | Data deposited in or computed by PubChem | |
Molecular Weight |
238.028 g/mol | |
Source | PubChem | |
URL | https://pubchem.ncbi.nlm.nih.gov | |
Description | Data deposited in or computed by PubChem | |
CAS No. |
12035-64-2 | |
Record name | Nickel phosphide (Ni2P) | |
Source | ChemIDplus | |
URL | https://pubchem.ncbi.nlm.nih.gov/substance/?source=chemidplus&sourceid=0012035642 | |
Description | ChemIDplus is a free, web search system that provides access to the structure and nomenclature authority files used for the identification of chemical substances cited in National Library of Medicine (NLM) databases, including the TOXNET system. | |
Record name | Dinickel phosphide | |
Source | European Chemicals Agency (ECHA) | |
URL | https://echa.europa.eu/substance-information/-/substanceinfo/100.031.649 | |
Description | The European Chemicals Agency (ECHA) is an agency of the European Union which is the driving force among regulatory authorities in implementing the EU's groundbreaking chemicals legislation for the benefit of human health and the environment as well as for innovation and competitiveness. | |
Explanation | Use of the information, documents and data from the ECHA website is subject to the terms and conditions of this Legal Notice, and subject to other binding limitations provided for under applicable law, the information, documents and data made available on the ECHA website may be reproduced, distributed and/or used, totally or in part, for non-commercial purposes provided that ECHA is acknowledged as the source: "Source: European Chemicals Agency, http://echa.europa.eu/". Such acknowledgement must be included in each copy of the material. ECHA permits and encourages organisations and individuals to create links to the ECHA website under the following cumulative conditions: Links can only be made to webpages that provide a link to the Legal Notice page. | |
Disclaimer and Information on In-Vitro Research Products
Please be aware that all articles and product information presented on BenchChem are intended solely for informational purposes. The products available for purchase on BenchChem are specifically designed for in-vitro studies, which are conducted outside of living organisms. In-vitro studies, derived from the Latin term "in glass," involve experiments performed in controlled laboratory settings using cells or tissues. It is important to note that these products are not categorized as medicines or drugs, and they have not received approval from the FDA for the prevention, treatment, or cure of any medical condition, ailment, or disease. We must emphasize that any form of bodily introduction of these products into humans or animals is strictly prohibited by law. It is essential to adhere to these guidelines to ensure compliance with legal and ethical standards in research and experimentation.