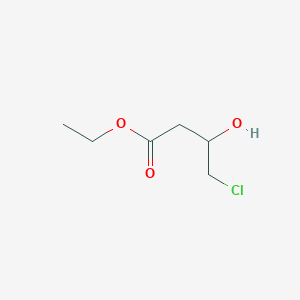
Ethyl 4-chloro-3-hydroxybutanoate
Overview
Description
Ethyl 4-chloro-3-hydroxybutanoate (CHBE) is a chiral ester with two enantiomers: (S)-CHBE and (R)-CHBE. It serves as a critical intermediate in synthesizing statins like atorvastatin (Lipitor®) and other pharmaceuticals targeting cholesterol and hypertension . The compound’s stereochemistry determines its application:
- (S)-CHBE: Primarily used in statin production due to its structural alignment with hydroxymethylglutaryl-CoA (HMG-CoA) reductase inhibitors .
- (R)-CHBE: Employed in antihypertensive agents (e.g., dilevalol) and other chiral drugs .
The synthesis of CHBE involves asymmetric reduction of ethyl 4-chloro-3-oxobutanoate (COBE) using biocatalysts such as carbonyl reductases, alcohol dehydrogenases, or whole-cell systems. Key challenges include achieving high enantiomeric excess (ee), yield, and scalability while minimizing cofactor dependency .
Preparation Methods
Synthetic Routes and Reaction Conditions: Ethyl 4-chloro-3-hydroxybutanoate can be synthesized through the asymmetric reduction of ethyl 4-chloro-3-oxobutanoate. This process often involves the use of biocatalysts such as carbonyl reductase and glucose dehydrogenase. The reaction typically occurs in an aqueous-organic solvent system, with optimized conditions to achieve high yield and enantiomeric excess .
Industrial Production Methods: In industrial settings, the production of this compound involves the use of recombinant Escherichia coli strains that express the necessary enzymes for the reduction process. The biocatalytic process is scaled up in fermentors, where conditions such as enzyme activity and cell biomass are carefully controlled to maximize production efficiency .
Chemical Reactions Analysis
Types of Reactions: Ethyl 4-chloro-3-hydroxybutanoate primarily undergoes reduction reactions. The asymmetric reduction of ethyl 4-chloro-3-oxobutanoate to this compound is a key reaction, often catalyzed by enzymes such as carbonyl reductase .
Common Reagents and Conditions: The reduction process typically involves the use of NADPH as a cofactor, with glucose serving as a hydrogen donor. The reaction conditions include a pH-buffered aqueous-organic solvent system, with temperatures maintained between 28 to 33°C .
Major Products: The primary product of these reactions is this compound itself, which is obtained with high yield and enantiomeric excess .
Scientific Research Applications
Pharmaceutical Applications
- Synthesis of Statins
- Chiral Building Block
- Immunosuppressive Agents
Biocatalytic Applications
The use of biocatalysts for the asymmetric synthesis of ECHB has gained attention due to its environmental benefits and efficiency:
- Enzymatic Synthesis : Recombinant Escherichia coli expressing specific alcohol dehydrogenases can convert ethyl 4-chloroacetoacetate into ECHB with high enantiomeric excess and conversion yield. This method showcases a sustainable approach to producing chiral compounds without toxic reagents .
Case Study 1: Synthesis via Recombinant Microorganisms
A study demonstrated that using recombinant E. coli cells expressing a secondary alcohol dehydrogenase resulted in a yield of 36.6 g/L of (R)-ECHB with over 99% enantiomeric excess. This method effectively regenerates NADH using 2-propanol as an energy source, highlighting its potential for industrial applications in producing chiral alcohols .
Case Study 2: Development of Atorvastatin
In another research initiative, ECHB was synthesized as part of a multi-step process to produce atorvastatin. The strategic incorporation of ECHB allowed for better control over stereochemistry, leading to improved efficacy and safety profiles for the final pharmaceutical product .
Data Table: Summary of Applications
Application Area | Specific Use | Notes |
---|---|---|
Pharmaceutical Synthesis | Atorvastatin | Key chiral intermediate |
Chiral Building Block | Synthesis of immunosuppressants | Used to prepare FR252921 |
Biocatalytic Processes | Enzymatic synthesis via recombinant bacteria | Sustainable method with high yields |
Mechanism of Action
The mechanism of action of ethyl 4-chloro-3-hydroxybutanoate involves its role as a chiral intermediate in enzymatic reactions. The compound is reduced from ethyl 4-chloro-3-oxobutanoate by carbonyl reductase, with NADPH acting as a cofactor. This reduction process is highly stereoselective, resulting in the production of enantiomerically pure this compound .
Comparison with Similar Compounds
Comparison with Similar Compounds
Comparison of (S)-CHBE and (R)-CHBE
The enantiomers of CHBE differ in their biocatalytic synthesis routes, stereoselectivity, and industrial applicability:
Key Findings:
- Cofactor Efficiency : Methods for (S)-CHBE often replace or eliminate external cofactors. For example, L-glutamine and glycine substitute NAD+ in β-cyclodextrin-water systems, achieving 98% yield and >99% ee . In contrast, (R)-CHBE synthesis typically relies on NADPH .
- Thermostability : Engineered ketoreductases (e.g., ChKRED20 mutant) enhance (S)-CHBE production under industrial conditions .
- Solvent Systems : Aqueous-organic diphasic systems improve substrate solubility for (R)-CHBE, while deep eutectic solvents (e.g., [ChCl][Gly]) optimize (S)-CHBE yields .
Comparison with Structurally Similar Compounds
CHBE shares functional similarities with other 3-hydroxybutanoate esters, but its chloro substituent enhances reactivity in drug synthesis:
Table 2: Comparison with Ethyl 3-Hydroxybutanoate Derivatives
Compound | Key Feature | Application | Synthesis Challenge |
---|---|---|---|
Ethyl (S)-4-chloro-3-hydroxybutanoate | Chloro group at C4 | Statins (atorvastatin) | High stereoselectivity required |
Ethyl (S)-3-hydroxy-3-phenylpropanoate | Phenyl group at C3 | Anticonvulsants, fragrances | Bulky substituent reduces yield |
Ethyl 3-hydroxybutanoate | No substituent | Biodegradable polymers | Low industrial demand |
- Ethyl (S)-3-hydroxy-3-phenylpropanoate: Synthesized via yeast-mediated reduction of ethyl benzoylacetate. While achieving >97% ee, its bulky phenyl group complicates large-scale production .
- Ethyl 3-hydroxybutanoate: Lacks the chloro group, making it less valuable in pharmaceutical contexts but useful in biodegradable plastics .
Biological Activity
Ethyl 4-chloro-3-hydroxybutanoate, also known as ethyl (S)-4-chloro-3-hydroxybutanoate or (S)-CHBE, is a significant chiral intermediate in the synthesis of various pharmaceuticals, particularly statins like atorvastatin. This compound exhibits notable biological activities, primarily due to its role as a substrate in enzymatic reactions and its potential therapeutic applications.
Synthesis and Enzymatic Activity
The synthesis of this compound has been extensively studied, particularly through biocatalytic methods involving recombinant Escherichia coli expressing specific enzymes. These methods often utilize carbonyl reductases and glucose dehydrogenases to facilitate the asymmetric reduction of ethyl 4-chloro-3-oxobutanoate (COBE) to (S)-CHBE.
Key Research Findings
- Biocatalytic Synthesis : A study demonstrated the successful production of (S)-CHBE with over 99% enantiomeric excess using E. coli transformants co-expressing carbonyl reductase from Pichia stipitis and glucose dehydrogenase from Bacillus megaterium. The system achieved a yield of 1,398 mM in an organic phase, marking a significant advancement in yield efficiency compared to previous methods .
- Yield Optimization : Another research highlighted an optimized biocatalytic process where a recombinant E. coli was cultivated in a 500-L fermentor, achieving a yield of 97.2% for (S)-CHBE with an enantiomeric excess of 99% after just four hours of reaction time . This study emphasized the scalability and efficiency of using engineered microorganisms for producing pharmaceutical intermediates.
- Reaction Conditions : The optimal conditions for synthesizing (S)-CHBE include maintaining the reaction at temperatures between 28°C to 33°C and utilizing specific concentrations of substrates and enzymes. For instance, the use of hydrophosphate as a buffering agent and glucose as a hydrogen donor significantly enhances the reaction's efficiency .
This compound's biological activity is closely linked to its structural properties, allowing it to act as an inhibitor for HMG-CoA reductase, an essential enzyme in cholesterol biosynthesis. This inhibition is crucial for developing statins, which are widely used to manage cholesterol levels and reduce cardiovascular disease risk.
Therapeutic Applications
- Cholesterol-Lowering Agents : As a precursor for statins, (S)-CHBE plays a vital role in synthesizing drugs that lower cholesterol levels in patients with hyperlipidemia. Statins work by competitively inhibiting HMG-CoA reductase, leading to decreased cholesterol synthesis and increased uptake of LDL cholesterol from the bloodstream.
- Potential Antitumor Activity : Preliminary studies suggest that compounds related to this compound may exhibit antitumor properties, although more research is needed to elucidate these effects fully.
Case Studies
Several case studies have demonstrated the compound's efficacy in various applications:
- Case Study 1 : A clinical trial investigated atorvastatin's effects on patients with high cholesterol levels, showing significant reductions in LDL cholesterol and overall cardiovascular risk .
- Case Study 2 : Research into alternative uses for statin derivatives indicated potential applications in cancer therapy due to their ability to modulate cellular signaling pathways involved in tumor growth .
Q & A
Basic Research Questions
Q. What are the standard biocatalytic methods for synthesizing enantiomerically pure (S)-CHBE?
The asymmetric reduction of ethyl 4-chloro-3-oxobutanoate (COBE) to (S)-CHBE is commonly achieved using recombinant Escherichia coli or yeast strains expressing carbonyl reductase (CR) and glucose dehydrogenase (GDH) for cofactor regeneration. For example, Candida magnoliae-derived CR produces (S)-CHBE with >99% enantiomeric excess (e.e.) under optimized conditions (pH 6.2, 5°C) . Whole-cell biocatalysts in aqueous-organic solvent systems (e.g., n-butyl acetate/water) enhance substrate solubility and product yield . Analytical methods like GC and HPLC with chiral columns (e.g., Chiralcel OB) are used to determine optical purity .
Q. How do reaction parameters influence the yield of CHBE in whole-cell biocatalysis?
Key parameters include:
- pH : Optimal activity for CR is typically near pH 6.0–7.0 .
- Temperature : Mild conditions (25–30°C) prevent enzyme denaturation .
- Substrate feeding : Fed-batch addition of COBE reduces substrate inhibition, achieving >90% molar yield .
- Cofactor regeneration : GDH-coupled systems with glucose sustain NADPH levels, improving turnover .
Q. What analytical techniques validate the enantiomeric purity of CHBE?
- Gas Chromatography (GC) : Quantifies COBE and CHBE concentrations .
- Chiral HPLC : Measures enantiomeric excess using columns like Chiralcel OB or OD-H .
- Polarimetry : Confirms optical rotation consistency with literature values (e.g., [α]D²⁵ = +20.5° for (S)-CHBE) .
Advanced Research Questions
Q. How can site-directed mutagenesis improve the enantioselectivity and efficiency of carbonyl reductases?
Mutagenesis targeting substrate-binding pockets enhances stereoselectivity. For example, the W28A and S209G mutations in Lodderomyces elongisporus AKR increased (R)-CHBE production to >99% e.e. and reduced Km by 40% . Computational modeling (e.g., WHAT IF software) guides residue selection for rational enzyme design .
Q. What strategies resolve contradictions in enantioselectivity outcomes across different biocatalytic systems?
Discrepancies arise from enzyme source and reaction additives. For instance:
- Candida magnoliae CR yields (S)-CHBE , while Sporobolomyces salmonicolor aldehyde reductase produces (R)-CHBE .
- Additives like allyl alcohol (5% v/v) shift baker’s yeast selectivity from (S)- to (R)-CHBE by modulating enzyme conformation . Systematic screening of reductases (e.g., via genome mining) and additive libraries can identify optimal conditions .
Q. How do biocompatible solvents enhance CHBE biosynthesis in biphasic systems?
Solvents like choline chloride/glycerol ([ChCl][Gly]) improve substrate solubility and enzyme stability. In 12.5% [ChCl][Gly]-water media, E. coli CCZU-T15 achieved 98% conversion of COBE to (S)-CHBE, with a space-time yield of 56.51 mmol/L/h . Solvent polarity also modulates water activity, reducing byproduct formation .
Q. What experimental designs address low NADPH availability in large-scale CHBE production?
- Cofactor recycling : Couple CR with GDH and glucose, achieving NADPH turnover numbers >10,000 .
- Engineered strains : Overexpress NAD kinase (pos5) in E. coli to boost intracellular NADPH pools .
- Chemoenzymatic systems : Use Zn²⁺-supplemented media to stabilize halohydrin dehalogenases for tandem reactions .
Q. Data Contradiction Analysis
Q. Why do studies report varying optimal pH values for carbonyl reductases?
Discrepancies stem from enzyme origin and assay conditions. For example:
- Candida magnoliae CR has optimal activity at pH 6.2 , while Pichia stipitis CR functions best at pH 7.5 .
- Ionic liquids (e.g., [Bmim]PF₆) can shift pH optima by altering enzyme microenvironments . Standardize assays in buffer-only systems to isolate pH effects.
Q. How can conflicting reports on substrate inhibition thresholds be reconciled?
Inhibition thresholds depend on biocatalyst form:
- Purified enzymes : COBE >50 mM inhibits CR due to active-site saturation .
- Whole cells : Intact membranes reduce free substrate concentration, allowing tolerances up to 200 mM COBE . Fed-batch substrate addition mitigates inhibition in both systems .
Q. Methodological Best Practices
Q. What protocols ensure reproducibility in CHBE biocatalysis?
- Strain validation : Use Sanger sequencing to confirm reductase gene integrity .
- Standardized analytics : Calibrate HPLC/GC with authentic (S)- and (R)-CHBE standards .
- Process controls : Monitor NADPH/NADH ratios via spectrophotometry (A₃₄₀) during reactions .
Q. How to scale up CHBE production without compromising enantiopurity?
Properties
IUPAC Name |
ethyl 4-chloro-3-hydroxybutanoate | |
---|---|---|
Source | PubChem | |
URL | https://pubchem.ncbi.nlm.nih.gov | |
Description | Data deposited in or computed by PubChem | |
InChI |
InChI=1S/C6H11ClO3/c1-2-10-6(9)3-5(8)4-7/h5,8H,2-4H2,1H3 | |
Source | PubChem | |
URL | https://pubchem.ncbi.nlm.nih.gov | |
Description | Data deposited in or computed by PubChem | |
InChI Key |
ZAJNMXDBJKCCAT-UHFFFAOYSA-N | |
Source | PubChem | |
URL | https://pubchem.ncbi.nlm.nih.gov | |
Description | Data deposited in or computed by PubChem | |
Canonical SMILES |
CCOC(=O)CC(CCl)O | |
Source | PubChem | |
URL | https://pubchem.ncbi.nlm.nih.gov | |
Description | Data deposited in or computed by PubChem | |
Molecular Formula |
C6H11ClO3 | |
Source | PubChem | |
URL | https://pubchem.ncbi.nlm.nih.gov | |
Description | Data deposited in or computed by PubChem | |
DSSTOX Substance ID |
DTXSID90402594 | |
Record name | ethyl 4-chloro-3-hydroxybutanoate | |
Source | EPA DSSTox | |
URL | https://comptox.epa.gov/dashboard/DTXSID90402594 | |
Description | DSSTox provides a high quality public chemistry resource for supporting improved predictive toxicology. | |
Molecular Weight |
166.60 g/mol | |
Source | PubChem | |
URL | https://pubchem.ncbi.nlm.nih.gov | |
Description | Data deposited in or computed by PubChem | |
CAS No. |
10488-69-4 | |
Record name | Butanoic acid, 4-chloro-3-hydroxy-, ethyl ester | |
Source | CAS Common Chemistry | |
URL | https://commonchemistry.cas.org/detail?cas_rn=10488-69-4 | |
Description | CAS Common Chemistry is an open community resource for accessing chemical information. Nearly 500,000 chemical substances from CAS REGISTRY cover areas of community interest, including common and frequently regulated chemicals, and those relevant to high school and undergraduate chemistry classes. This chemical information, curated by our expert scientists, is provided in alignment with our mission as a division of the American Chemical Society. | |
Explanation | The data from CAS Common Chemistry is provided under a CC-BY-NC 4.0 license, unless otherwise stated. | |
Record name | ethyl 4-chloro-3-hydroxybutanoate | |
Source | EPA DSSTox | |
URL | https://comptox.epa.gov/dashboard/DTXSID90402594 | |
Description | DSSTox provides a high quality public chemistry resource for supporting improved predictive toxicology. | |
Record name | ethyl 4-chloro-3-hydroxybutanoate | |
Source | European Chemicals Agency (ECHA) | |
URL | https://echa.europa.eu/information-on-chemicals | |
Description | The European Chemicals Agency (ECHA) is an agency of the European Union which is the driving force among regulatory authorities in implementing the EU's groundbreaking chemicals legislation for the benefit of human health and the environment as well as for innovation and competitiveness. | |
Explanation | Use of the information, documents and data from the ECHA website is subject to the terms and conditions of this Legal Notice, and subject to other binding limitations provided for under applicable law, the information, documents and data made available on the ECHA website may be reproduced, distributed and/or used, totally or in part, for non-commercial purposes provided that ECHA is acknowledged as the source: "Source: European Chemicals Agency, http://echa.europa.eu/". Such acknowledgement must be included in each copy of the material. ECHA permits and encourages organisations and individuals to create links to the ECHA website under the following cumulative conditions: Links can only be made to webpages that provide a link to the Legal Notice page. | |
Synthesis routes and methods I
Procedure details
Synthesis routes and methods II
Procedure details
Synthesis routes and methods III
Procedure details
Synthesis routes and methods IV
Procedure details
Retrosynthesis Analysis
AI-Powered Synthesis Planning: Our tool employs the Template_relevance Pistachio, Template_relevance Bkms_metabolic, Template_relevance Pistachio_ringbreaker, Template_relevance Reaxys, Template_relevance Reaxys_biocatalysis model, leveraging a vast database of chemical reactions to predict feasible synthetic routes.
One-Step Synthesis Focus: Specifically designed for one-step synthesis, it provides concise and direct routes for your target compounds, streamlining the synthesis process.
Accurate Predictions: Utilizing the extensive PISTACHIO, BKMS_METABOLIC, PISTACHIO_RINGBREAKER, REAXYS, REAXYS_BIOCATALYSIS database, our tool offers high-accuracy predictions, reflecting the latest in chemical research and data.
Strategy Settings
Precursor scoring | Relevance Heuristic |
---|---|
Min. plausibility | 0.01 |
Model | Template_relevance |
Template Set | Pistachio/Bkms_metabolic/Pistachio_ringbreaker/Reaxys/Reaxys_biocatalysis |
Top-N result to add to graph | 6 |
Feasible Synthetic Routes
Disclaimer and Information on In-Vitro Research Products
Please be aware that all articles and product information presented on BenchChem are intended solely for informational purposes. The products available for purchase on BenchChem are specifically designed for in-vitro studies, which are conducted outside of living organisms. In-vitro studies, derived from the Latin term "in glass," involve experiments performed in controlled laboratory settings using cells or tissues. It is important to note that these products are not categorized as medicines or drugs, and they have not received approval from the FDA for the prevention, treatment, or cure of any medical condition, ailment, or disease. We must emphasize that any form of bodily introduction of these products into humans or animals is strictly prohibited by law. It is essential to adhere to these guidelines to ensure compliance with legal and ethical standards in research and experimentation.