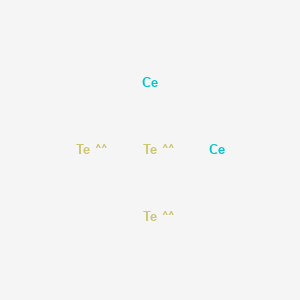
Cerium telluride
- Click on QUICK INQUIRY to receive a quote from our team of experts.
- With the quality product at a COMPETITIVE price, you can focus more on your research.
Overview
Description
Cerium telluride is a chemical compound composed of cerium and telluriumThis compound typically exists in the form of CeTe3, which has an orthorhombic crystal structure .
Preparation Methods
Synthetic Routes and Reaction Conditions: Cerium telluride can be synthesized through various methods, including solid-state reactions and chemical vapor transport. One common method involves the direct reaction of cerium and tellurium at high temperatures. The reaction is typically carried out in a sealed quartz tube under an inert atmosphere to prevent oxidation. The reaction conditions often include temperatures ranging from 600°C to 800°C .
Industrial Production Methods: In industrial settings, this compound can be produced using similar high-temperature methods. The process involves the careful control of reaction conditions to ensure the purity and quality of the final product. Industrial production may also involve the use of advanced techniques such as molten salt electrolysis and metal thermal reduction to achieve high purity levels .
Chemical Reactions Analysis
Types of Reactions: Cerium telluride undergoes various chemical reactions, including oxidation, reduction, and substitution reactions.
Common Reagents and Conditions:
Oxidation: this compound can be oxidized to form cerium oxide and tellurium dioxide. This reaction typically occurs in the presence of oxygen at elevated temperatures.
Reduction: this compound can be reduced using strong reducing agents such as hydrogen gas. This reaction is often carried out at high temperatures.
Substitution: this compound can undergo substitution reactions with halogens to form cerium halides and tellurium halides.
Major Products Formed:
Oxidation: Cerium oxide (CeO2) and tellurium dioxide (TeO2).
Reduction: Cerium metal and tellurium metal.
Substitution: Cerium halides (e.g., CeCl3) and tellurium halides (e.g., TeCl4).
Scientific Research Applications
Cerium telluride has a wide range of scientific research applications due to its unique properties:
Chemistry:
- Used as a semiconductor material in various electronic devices.
- Employed in the synthesis of other tellurium-based compounds.
Biology:
- Investigated for its potential use in biosensors and bioimaging due to its semiconducting properties.
Medicine:
- Explored for its potential use in drug delivery systems and as a contrast agent in medical imaging.
Industry:
- Utilized in the production of thermoelectric materials for energy conversion.
- Applied in the development of advanced materials for optoelectronic devices .
Mechanism of Action
The mechanism of action of cerium telluride is primarily related to its semiconducting properties. It can interact with various molecular targets and pathways, depending on its application. For example, in electronic devices, this compound can facilitate the flow of electrons, thereby enhancing the device’s performance. In biological applications, this compound can interact with cellular components, potentially affecting cellular processes and signaling pathways .
Comparison with Similar Compounds
Cadmium telluride (CdTe): A widely used semiconductor material in solar cells and optoelectronic devices.
Bismuth telluride (Bi2Te3): Known for its thermoelectric properties and used in thermoelectric generators.
Cerium oxide (CeO2): Used in catalytic converters and as a polishing agent
Uniqueness: Cerium telluride is unique due to its combination of cerium and tellurium, which imparts distinct semiconducting properties. Unlike cadmium telluride and bismuth telluride, this compound offers a different set of electronic and optical properties, making it suitable for specific applications in electronics and materials science .
Properties
InChI |
InChI=1S/2Ce.3Te |
Source
|
---|---|---|
Source | PubChem | |
URL | https://pubchem.ncbi.nlm.nih.gov | |
Description | Data deposited in or computed by PubChem | |
InChI Key |
KMYDRZUGLSMFOC-UHFFFAOYSA-N |
Source
|
Source | PubChem | |
URL | https://pubchem.ncbi.nlm.nih.gov | |
Description | Data deposited in or computed by PubChem | |
Canonical SMILES |
[Te].[Te].[Te].[Ce].[Ce] |
Source
|
Source | PubChem | |
URL | https://pubchem.ncbi.nlm.nih.gov | |
Description | Data deposited in or computed by PubChem | |
Molecular Formula |
Ce2Te3 |
Source
|
Source | PubChem | |
URL | https://pubchem.ncbi.nlm.nih.gov | |
Description | Data deposited in or computed by PubChem | |
Molecular Weight |
663.0 g/mol |
Source
|
Source | PubChem | |
URL | https://pubchem.ncbi.nlm.nih.gov | |
Description | Data deposited in or computed by PubChem | |
CAS No. |
12014-97-0 |
Source
|
Record name | Cerium telluride (Ce2Te3) | |
Source | ChemIDplus | |
URL | https://pubchem.ncbi.nlm.nih.gov/substance/?source=chemidplus&sourceid=0012014970 | |
Description | ChemIDplus is a free, web search system that provides access to the structure and nomenclature authority files used for the identification of chemical substances cited in National Library of Medicine (NLM) databases, including the TOXNET system. | |
Record name | Cerium telluride (Ce2Te3) | |
Source | EPA Chemicals under the TSCA | |
URL | https://www.epa.gov/chemicals-under-tsca | |
Description | EPA Chemicals under the Toxic Substances Control Act (TSCA) collection contains information on chemicals and their regulations under TSCA, including non-confidential content from the TSCA Chemical Substance Inventory and Chemical Data Reporting. | |
Record name | Dicerium tritelluride | |
Source | European Chemicals Agency (ECHA) | |
URL | https://echa.europa.eu/substance-information/-/substanceinfo/100.031.447 | |
Description | The European Chemicals Agency (ECHA) is an agency of the European Union which is the driving force among regulatory authorities in implementing the EU's groundbreaking chemicals legislation for the benefit of human health and the environment as well as for innovation and competitiveness. | |
Explanation | Use of the information, documents and data from the ECHA website is subject to the terms and conditions of this Legal Notice, and subject to other binding limitations provided for under applicable law, the information, documents and data made available on the ECHA website may be reproduced, distributed and/or used, totally or in part, for non-commercial purposes provided that ECHA is acknowledged as the source: "Source: European Chemicals Agency, http://echa.europa.eu/". Such acknowledgement must be included in each copy of the material. ECHA permits and encourages organisations and individuals to create links to the ECHA website under the following cumulative conditions: Links can only be made to webpages that provide a link to the Legal Notice page. | |
Disclaimer and Information on In-Vitro Research Products
Please be aware that all articles and product information presented on BenchChem are intended solely for informational purposes. The products available for purchase on BenchChem are specifically designed for in-vitro studies, which are conducted outside of living organisms. In-vitro studies, derived from the Latin term "in glass," involve experiments performed in controlled laboratory settings using cells or tissues. It is important to note that these products are not categorized as medicines or drugs, and they have not received approval from the FDA for the prevention, treatment, or cure of any medical condition, ailment, or disease. We must emphasize that any form of bodily introduction of these products into humans or animals is strictly prohibited by law. It is essential to adhere to these guidelines to ensure compliance with legal and ethical standards in research and experimentation.