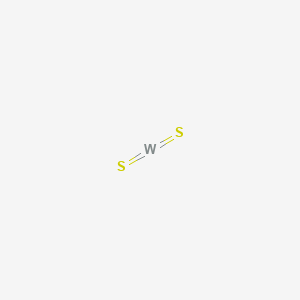
Tungsten disulfide
- Click on QUICK INQUIRY to receive a quote from our team of experts.
- With the quality product at a COMPETITIVE price, you can focus more on your research.
Overview
Description
Tungsten disulfide is an inorganic compound composed of tungsten and sulfur, with the chemical formula WS₂. It belongs to the family of transition metal dichalcogenides and occurs naturally as the rare mineral tungstenite . This compound is known for its layered structure, which imparts unique properties such as high lubricity and thermal stability. This compound is widely used in various industrial applications, including lubricants, catalysts, and energy storage devices .
Mechanism of Action
Biological Membranes: WS₂ nanosheets adhere to bacterial surfaces, leading to robust inhibition of cell proliferation. Once a bacterium is fully covered with WS₂, its growth is significantly retarded .
Electrode Materials: WS₂ is used as an electrode material in energy storage and conversion devices. Its unique properties make it a competent substitute for next-generation environmentally benign energy storage systems .
Mode of Action:
WS₂ exerts its effects through several mechanisms:
Antibacterial Activity: WS₂ nanosheets adhere to bacterial surfaces, disrupting cell membranes and inhibiting cell proliferation. This membrane destruction-mediated antibacterial activity is concentration-dependent .
Electrocatalysis: WS₂ demonstrates significant effectiveness in the hydrogen-evolution reaction (HER) due to its electrocatalytic properties .
Biochemical Pathways:
WS₂ affects various pathways, including:
- Energy Storage and Conversion : WS₂-based composites contribute to clean energy and high-efficiency energy conservation by optimizing electrode materials in electrochemical energy storage systems .
Action Environment:
Environmental factors, such as temperature, humidity, and surface properties, influence WS₂ stability, efficacy, and performance. For instance, WS₂ coatings can be used at elevated temperatures in outer space .
Biochemical Analysis
Biochemical Properties
Tungsten disulfide interacts with various biomolecules in biochemical reactions. It possesses the ability to absorb near-infrared light, specifically within the range of 700–1100 nm, and displays exceptional optical stability and an impressive photothermal conversion efficiency . This makes this compound an ideal candidate for imaging-guided anti-tumor photothermal therapy .
Cellular Effects
This compound has shown significant effects on various types of cells and cellular processes. For instance, it has demonstrated antibacterial activities against two representative bacterial strains: Gram-negative Escherichia coli (E. coli) and Gram-positive Staphylococcus aureus (S. aureus) .
Molecular Mechanism
The molecular mechanism of action of this compound is complex and multifaceted. It exerts its effects at the molecular level through various interactions with biomolecules, including binding interactions, enzyme inhibition or activation, and changes in gene expression .
Temporal Effects in Laboratory Settings
The effects of this compound change over time in laboratory settings. Information on the product’s stability, degradation, and any long-term effects on cellular function observed in in vitro or in vivo studies is currently being researched .
Dosage Effects in Animal Models
The effects of this compound vary with different dosages in animal models. Research is ongoing to determine any threshold effects observed in these studies, as well as any toxic or adverse effects at high doses .
Metabolic Pathways
This compound is involved in various metabolic pathways. It interacts with several enzymes or cofactors, and may have effects on metabolic flux or metabolite levels .
Subcellular Localization
The subcellular localization of this compound and any effects on its activity or function are areas of active research .
Preparation Methods
Synthetic Routes and Reaction Conditions: Tungsten disulfide can be synthesized through several methods, including:
Chemical Vapor Deposition (CVD): This method involves the reaction of tungsten hexacarbonyl (W(CO)₆) with hydrogen sulfide (H₂S) at high temperatures to form this compound.
Hydrothermal/Solvothermal Method: This involves the reaction of tungsten precursors such as sodium tungstate (Na₂WO₄) with sulfur sources like thiourea (CH₄N₂S) under high temperature and pressure in a sealed system.
Liquid Phase Synthesis: this compound can also be prepared by reacting tungsten chloride (WCl₆) with sulfur in a liquid medium.
Industrial Production Methods: Industrial production of this compound typically involves large-scale methods such as:
Colloidal Precipitation: This method involves the sulfurization of tungsten oxide (WO₃) using hydrogen sulfide (H₂S) or other sulfur sources, followed by calcination to obtain this compound.
Mechanical Exfoliation: Bulk this compound is exfoliated into nanosheets using mechanical forces, such as high-intensity ultrasound.
Types of Reactions:
Reduction: this compound can be reduced to tungsten metal (W) using reducing agents such as hydrogen gas (H₂).
Common Reagents and Conditions:
Oxidation: Requires oxygen (O₂) and water (H₂O) under ambient light and atmospheric conditions.
Reduction: Requires hydrogen gas (H₂) at elevated temperatures.
Substitution: Requires chalcogen sources like selenium (Se) or tellurium (Te) under controlled conditions.
Major Products:
Oxidation: Tungsten oxide (WO₃) and sulfuric acid (H₂SO₄).
Reduction: Tungsten metal (W).
Substitution: Tungsten diselenide (WSe₂) or tungsten ditelluride (WTe₂).
Scientific Research Applications
Tungsten disulfide has a wide range of scientific research applications, including:
Comparison with Similar Compounds
Tungsten disulfide is often compared with other transition metal dichalcogenides, such as:
Molybdenum disulfide (MoS₂): Similar layered structure and properties, but this compound has higher thermal stability and lubricity.
Tungsten diselenide (WSe₂): Similar structure but different electronic properties due to the presence of selenium instead of sulfur.
Tungsten ditelluride (WTe₂): Exhibits unique topological properties not found in this compound.
These comparisons highlight the uniqueness of this compound in terms of its thermal stability, lubricity, and electronic properties.
Biological Activity
Tungsten disulfide (WS₂) is a transition metal dichalcogenide that has garnered significant attention in recent years due to its unique properties and potential applications in various fields, including biomedicine. This article provides a comprehensive overview of the biological activity of WS₂, focusing on its antibacterial properties, biocompatibility, and potential therapeutic applications.
Antibacterial Activity
Recent studies have demonstrated the antibacterial properties of WS₂ nanosheets against both Gram-negative Escherichia coli and Gram-positive Staphylococcus aureus. The antibacterial mechanism primarily involves:
- Membrane Disruption : WS₂ nanosheets adhere to bacterial surfaces, leading to significant damage to the cell membrane. This results in cell death due to compromised membrane integrity.
- Reactive Oxygen Species (ROS) : Although WS₂ generates ROS, their levels are modest compared to other materials like molybdenum disulfide (MoS₂), indicating that membrane damage is a more critical factor in its antibacterial action .
Table 1: Antibacterial Efficacy of WS₂ Nanosheets
Bacterial Strain | Concentration (µg/mL) | Growth Inhibition (%) | Mechanism |
---|---|---|---|
E. coli | 10 | 70 | Membrane disruption |
S. aureus | 10 | 65 | Membrane disruption |
E. coli | 50 | 90 | Membrane disruption |
S. aureus | 50 | 85 | Membrane disruption |
Biocompatibility
The biocompatibility of WS₂ has been assessed through various in vitro and in vivo studies. Notably, research involving WS₂ nanotubes and fullerene-like nanoparticles showed no significant cytotoxic effects on human salivary gland cells and rat submandibular cells. Key findings include:
- Cell Viability : Growth curves and viability tests indicated over 90% viability across various concentrations of WS₂ nanoparticles.
- Morphological Integrity : No significant alterations in cell morphology were observed when exposed to WS₂, suggesting favorable interactions with biological tissues .
Table 2: Biocompatibility Assessment of WS₂ Nanoparticles
Cell Type | Concentration (µg/mL) | Viability (%) | Morphological Changes |
---|---|---|---|
Salivary Gland Cells | 0.22 | >90 | None |
Rat Submandibular Cells | 35.2 | >90 | None |
Potential Therapeutic Applications
Given its unique properties, WS₂ has potential applications in drug delivery systems and as a therapeutic agent. Some promising avenues include:
- Drug Delivery : The ability of WS₂ to be functionalized for specific targeting enhances its utility in delivering therapeutic agents directly to diseased tissues.
- Electrocatalysis : WS₂ shows promise as an electrocatalyst for hydrogen evolution reactions, which could be beneficial in energy-related applications .
- Regenerative Medicine : Studies indicate that WS₂ can support the proliferation and differentiation of mesenchymal stem cells, making it a candidate for tissue engineering applications .
Case Studies
-
Antibacterial Efficacy Study :
A study evaluated the antibacterial effects of WS₂ nanosheets against E. coli and S. aureus, demonstrating significant growth inhibition through membrane destruction mechanisms . -
Biocompatibility Study :
In vitro experiments on rat submandibular cells revealed that WS₂ nanoparticles did not induce cytotoxicity or morphological changes, supporting their use in biomedical applications . -
Therapeutic Application Research :
Research into the use of WS₂ as a drug delivery vehicle showed that it can effectively encapsulate drugs while maintaining low toxicity levels, indicating its potential for targeted therapy .
Properties
CAS No. |
12138-09-9 |
---|---|
Molecular Formula |
S2W-4 |
Molecular Weight |
248.0 g/mol |
IUPAC Name |
tungsten;disulfide |
InChI |
InChI=1S/2S.W/q2*-2; |
InChI Key |
OWRBHDYZJXBLLF-UHFFFAOYSA-N |
SMILES |
S=[W]=S |
Canonical SMILES |
[S-2].[S-2].[W] |
Key on ui other cas no. |
12138-09-9 |
physical_description |
OtherSolid |
Pictograms |
Irritant |
Origin of Product |
United States |
Q1: What is the molecular formula and weight of tungsten disulfide?
A1: The molecular formula of this compound is WS2. Its molecular weight is 247.98 g/mol.
Q2: What is the crystal structure of this compound?
A2: this compound exhibits a layered structure similar to molybdenum disulfide, with each layer composed of a plane of tungsten atoms sandwiched between two planes of sulfur atoms. []
Q3: What are the characteristic Raman modes of WS2?
A3: WS2 exhibits three characteristic Raman modes: E2g, A1g, and 2LA. The E2g and A1g modes arise from in-plane stretching optical phonon modes, while the 2LA mode comes from a longitudinal acoustic mode. []
Q4: How does the presence of oxygen affect WS2 monolayers?
A4: WS2 monolayers are susceptible to oxidation under ambient conditions, leading to degradation. This degradation is significantly reduced when WS2 is grown on graphene substrates due to the screening effect of the surface electric field created by graphene. []
Q5: How does the choice of substrate impact the stability and properties of WS2 monolayers?
A5: The substrate plays a crucial role in modulating the properties of WS2 monolayers. For instance, WS2 on SiO2 substrates oxidizes within weeks, while WS2 on suspended graphene exhibits enhanced oxidation resistance. [] Additionally, using two-layer graphene as a substrate for WS2 monolayers leads to a significant enhancement of the photoluminescence response compared to WS2 supported on one-layer graphene. []
Q6: How does the addition of this compound affect the electrical conductivity of copper matrix composites?
A7: While this compound itself has insulating properties, incorporating it into copper matrix composites (CuMCs) results in only a slight decrease in electrical conductivity compared to pure copper. This makes CuMCs with WS2 suitable for applications where both conductivity and lubrication are crucial. []
Q7: Can this compound be used as a solid lubricant in space applications?
A8: Yes, this compound is a suitable solid lubricant for space environments because it can withstand low pressures and wide temperature variations. Research has shown it can exhibit lower friction coefficients than other solid lubricants like gold film and molybdenum disulfide in simulated space environments. []
Q8: How does this compound improve the performance of foil bearings operating under dry conditions?
A9: this compound-based solid lubricants, when applied to foil pads in gas foil bearings, exhibit excellent performance, particularly when paired with hard, wear-resistant coatings like chrome plating or hydrogenated diamond-like carbon (H-DLC) on the rotating disks. The soft solid lubricant coating on the foil pad and the hard coating on the disk minimize friction and wear, promoting reliable operation. []
Q9: How is this compound used in the development of water-based lubricants?
A10: Nanometer-sized this compound is a key component in water-based lubricants, contributing to their improved lubrication properties and higher elongation coefficients. These lubricants are environmentally friendly and have a wide range of applications due to their excellent performance. []
Q10: How is the performance of this compound as a solid lubricant affected by its particle size?
A11: The particle size of this compound plays a significant role in its lubrication properties. Studies using spherical this compound particles with average diameters ranging from 5 to 50 micrometers show improved performance in reducing friction. [] Similarly, this compound nanorods with diameters of 10-25 nm and lengths of 1-5 μm exhibit enhanced anti-friction properties and oxidation resistance. []
Q11: Can this compound be used as a catalyst for hydrogen evolution?
A12: Yes, silica-supported this compound (WS2/SiO2) exhibits promising catalytic activity for hydrogen evolution, comparable to and even surpassing platinum-based catalysts in certain aspects. It demonstrates activity both in the dark and under visible light illumination when paired with a suitable sensitizer like cadmium sulfide. []
Q12: What are some notable nanostructures of this compound and their applications?
A12: this compound can be synthesized into various nanostructures, each with unique properties. For instance:
- This compound nanosheets are utilized in high-performance quasi-solid-state asymmetric supercapacitors due to their high capacitance and conductivity. []
- This compound nanofibers, with diameters under 100 nm and lengths exceeding 10 mm, have potential applications in electronics and catalysis. []
- This compound nanorods are explored as friction-reducing additives in lubricants. []
Q13: How do this compound nanosheets enhance the performance of supercapacitors?
A14: When immobilized on active carbon fibers, this compound nanosheets create a nanocomposite with a high surface area, facilitating greater ion storage. The nanosheets provide numerous active sites for electrochemical reactions, leading to a high specific capacitance. Moreover, the interwoven structure with the conductive carbon fibers enhances electron transport, leading to high power density. []
Q14: How does the morphology of this compound nanosheets impact their properties and applications?
A15: The morphology of WS2 nanosheets significantly influences their properties. For example, controlling the reaction time during solvothermal synthesis can alter the number of atomic layers in the nanosheets, affecting their electronic and optical properties. []
Q15: How is this compound being explored for use in biosensors?
A16: this compound's unique electrochemical properties make it suitable for biosensing applications. For instance, it has been successfully employed in developing a paper-based electrochemical sensor for detecting Listeria monocytogenes. This sensor utilizes a WS2/aptamer hybrid on an electrochemical paper-based analytical device (ePAD), demonstrating its potential for food safety applications. []
Q16: How are this compound and reduced graphene oxide combined for thermoelectric applications?
A17: Hybridizing this compound with reduced graphene oxide (rGO) creates a composite material with enhanced thermoelectric properties. While WS2 exhibits a high Seebeck coefficient, its low electrical conductivity limits its thermoelectric performance. The addition of highly conductive rGO addresses this limitation by creating efficient pathways for charge carrier transport. []
Q17: How do excitonic effects in WS2 monolayers change when placed on different layers of graphene?
A18: The photoluminescence (PL) response of WS2 monolayers is significantly influenced by the number of graphene layers underneath. This is attributed to the layer-dependent charge transfer at the interface between WS2 and graphene, influencing the formation and recombination of excitons in WS2. []
Q18: What role does this compound play in the development of advanced lasers?
A19: this compound's unique optical properties make it suitable for various laser applications. For example, it has been successfully employed as a saturable absorber in Q-switched erbium-doped fiber lasers, leading to pulsed laser operation with tunable repetition rates. [, ] Moreover, WS2-based photonic crystal surface-emitting lasers operating at room temperature have been demonstrated, showcasing the potential for compact and efficient light sources. []
Q19: Can you explain the significance of spin-valley coupling in this compound for optical applications?
A20: this compound exhibits strong spin-valley coupling, allowing for the selective excitation of excitons in specific valleys using circularly polarized light. This property has significant implications for developing valleytronic devices, where information can be encoded and manipulated using the valley degree of freedom. []
Q20: How does chemical doping affect the optical properties of monolayer this compound?
A21: Chemical doping offers a viable approach for modulating the optical properties of monolayer WS2. For example, doping with specific chemical agents can lead to significant enhancement of photoluminescence, primarily by reducing the formation of electron-bound trions that typically decrease PL efficiency. []
Q21: Can this compound be used for sensing applications beyond biosensing?
A22: Yes, the unique properties of this compound extend its applications to various sensing scenarios. For example, coating tapered optical fibers with WS2 significantly enhances their sensitivity for detecting urea in clinical samples. This improvement stems from the synergistic combination of WS2's optoelectronic properties and the light-guiding characteristics of the tapered fiber. []
Q22: What are some common methods for preparing this compound thin films?
A22: this compound thin films can be prepared using various techniques, including:
- Magnetron sputtering: This method allows for controlled deposition of WS2 films, but directly sputtered films often exhibit low photoactivity due to defects like sulfur vacancies. []
- Two-step processes: These involve depositing a sulfur-rich tungsten sulfide precursor at low temperatures followed by annealing in a sulfur-containing atmosphere. This approach has shown promise in producing photoactive WS2 films. []
Q23: How does the preparation method of this compound affect its properties?
A24: The method used to prepare WS2 significantly impacts its characteristics. For instance, while direct magnetron sputtering of WS2 can yield films with good structural quality, they might lack photoactivity due to defects introduced during the process. [] Employing a two-step method, involving the deposition of a precursor followed by annealing, can overcome this limitation and produce photoactive WS2 films. []
Q24: How does the thermal decomposition of ammonium tetrathiotungstate lead to the formation of this compound nanofibers?
A25: The thermal decomposition of ammonium tetrathiotungstate provides a controlled route for synthesizing WS2 nanofibers. By carefully adjusting parameters like heating rate, cooling rate, atmosphere, and reactant size during the decomposition process, the growth of these nanofibers can be precisely controlled. []
Q25: How can the properties of this compound be further tailored for specific applications?
A25: Ongoing research focuses on fine-tuning the properties of WS2 through various strategies, including:
- Doping: Introducing impurities like titanium can alter the electronic and catalytic properties of WS2. []
- Functionalization: Modifying WS2 with metal oxides like iron oxide or nickel oxide can enhance its performance in applications like flame retardants and nanocomposites. []
- Heterostructure formation: Combining WS2 with other 2D materials, such as graphene or black phosphorus, can create heterostructures with novel properties and functionalities. []
Disclaimer and Information on In-Vitro Research Products
Please be aware that all articles and product information presented on BenchChem are intended solely for informational purposes. The products available for purchase on BenchChem are specifically designed for in-vitro studies, which are conducted outside of living organisms. In-vitro studies, derived from the Latin term "in glass," involve experiments performed in controlled laboratory settings using cells or tissues. It is important to note that these products are not categorized as medicines or drugs, and they have not received approval from the FDA for the prevention, treatment, or cure of any medical condition, ailment, or disease. We must emphasize that any form of bodily introduction of these products into humans or animals is strictly prohibited by law. It is essential to adhere to these guidelines to ensure compliance with legal and ethical standards in research and experimentation.