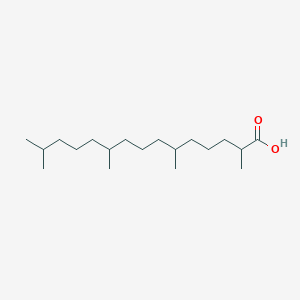
Pristanic acid
Overview
Description
Pristanic acid, also known as 2,6,10,14-tetramethylpentadecanoic acid, is a branched-chain fatty acid. It is a terpenoid acid present at micromolar concentrations in the blood plasma of healthy individuals. This compound is found in various lipids from sources such as freshwater sponges, krill, earthworms, whales, human milk fat, bovine depot fat, butterfat, and even Californian petroleum . It is usually present in combination with phytanic acid and can be obtained directly from the diet or as the alpha oxidation product of phytanic acid .
Mechanism of Action
Target of Action
Pristanic acid, a terpenoid acid, is a natural ligand for the peroxisome proliferator-activated receptor alpha (PPARα) . PPARα plays a crucial role in the regulation of lipid metabolism and inflammation .
Mode of Action
At physiological concentrations, this compound interacts with PPARα, activating this receptor . This interaction triggers a series of changes in gene expression that regulate lipid metabolism, inflammation, and other processes .
Biochemical Pathways
This compound is involved in the peroxisomal beta-oxidation pathway . In the liver, this compound is degraded by peroxisomal beta oxidation to propionyl-CoA . This process is essential for the breakdown of certain types of fatty acids, including this compound .
Pharmacokinetics
This compound is obtained from two sources in humans: directly from the diet or as the alpha oxidation product of phytanic acid . It is present at micromolar concentrations in the blood plasma of healthy individuals . The compound’s bioavailability is influenced by dietary intake and metabolic processes .
Result of Action
The activation of PPARα by this compound leads to changes in lipid metabolism and inflammation . In certain inherited disorders such as zellweger syndrome, this compound accumulates, leading to disease symptoms .
Action Environment
The action of this compound is influenced by various environmental factors. For instance, dietary intake can affect the levels of this compound in the body . Furthermore, certain genetic disorders can impair the body’s ability to metabolize this compound, leading to its accumulation and associated health problems .
Biochemical Analysis
Biochemical Properties
Pristanic acid plays a significant role in biochemical reactions. It is a natural ligand for peroxisome proliferator-activated receptor alpha (PPARα), a key regulator of lipid metabolism . This compound interacts with enzymes involved in its degradation, such as peroxisomal beta oxidation enzymes, which convert it to propionyl-CoA .
Cellular Effects
This compound influences cell function by modulating lipid metabolism through its interaction with PPARα . It can affect cell signaling pathways related to lipid homeostasis, influence gene expression patterns associated with fatty acid metabolism, and alter cellular metabolic processes .
Molecular Mechanism
At the molecular level, this compound exerts its effects through binding interactions with biomolecules like PPARα . It can activate or inhibit enzymes involved in its metabolism, leading to changes in gene expression related to lipid metabolism .
Metabolic Pathways
This compound is involved in the alpha oxidation pathway, where it is converted to propionyl-CoA by peroxisomal beta oxidation . This process involves various enzymes and cofactors, and can affect metabolic flux or metabolite levels.
Subcellular Localization
It is known that this compound is metabolized in peroxisomes
Preparation Methods
Pristanic acid can be synthesized through the alpha oxidation of phytanic acid. This process involves the conversion of phytanic acid to this compound via intermediates such as 2-hydroxy-phytanoyl-CoA and pristanal . Industrial production methods for this compound typically involve the extraction and purification from natural sources such as butterfat and other lipid-rich materials .
Chemical Reactions Analysis
Pristanic acid undergoes several types of chemical reactions, including:
Oxidation: This compound is degraded by peroxisomal beta oxidation to propionyl-CoA.
Reduction: While specific reduction reactions of this compound are less documented, it can potentially undergo reduction like other fatty acids.
Common reagents and conditions used in these reactions include enzymes involved in peroxisomal beta oxidation and various catalysts for esterification reactions. Major products formed from these reactions include propionyl-CoA and pristanates .
Scientific Research Applications
Comparison with Similar Compounds
Pristanic acid is similar to other branched-chain fatty acids such as phytanic acid, 4,8,12-trimethyltridecanoic acid, and 4,8-dimethylnonanoic acid . this compound is unique due to its specific role as a ligand for PPARα and its involvement in peroxisomal beta oxidation . Phytanic acid, for example, undergoes alpha oxidation to form this compound, highlighting the metabolic relationship between these compounds .
Biological Activity
Pristanic acid, also known as 2,6,10,14-tetramethylpentadecanoic acid, is a branched-chain fatty acid that plays a significant role in human metabolism, particularly in the context of peroxisomal disorders. This article explores the biological activity of this compound, highlighting its metabolic pathways, associations with diseases, and potential therapeutic implications.
Metabolism of this compound
This compound is primarily derived from the dietary intake of phytanic acid, which undergoes α-oxidation to form this compound. This metabolic process is crucial for the degradation of phytanic acid, especially in the context of peroxisomal metabolism. The enzyme α-Methyl-CoA-Racemase (AMACR) is instrumental in this pathway and has been linked to various pathological conditions, including prostate cancer.
Key Metabolic Pathways
- α-Oxidation : this compound is produced from phytanic acid through α-oxidation. This process occurs predominantly in peroxisomes and is essential for the proper metabolism of branched-chain fatty acids.
- β-Oxidation : Following its formation, this compound undergoes β-oxidation within peroxisomes. Studies indicate that this compound can be oxidized through three cycles of β-oxidation, leading to the generation of acetyl-CoA and other metabolites .
Biological Significance
This compound levels in plasma can serve as biomarkers for various metabolic disorders. Elevated levels are often observed in patients with peroxisomal biogenesis disorders (PBDs), such as Zellweger syndrome. The oxidation of this compound is significantly reduced in these patients, indicating impaired metabolic function .
Case Studies
- Peroxisomal Biogenesis Disorders : A study involving fibroblast cell lines from patients with PBDs demonstrated reduced oxidation rates of this compound compared to control cells. The findings suggest that measuring this compound oxidation can be a reliable tool for assessing metabolic function in these patients .
- Prostate Cancer Association : Research has shown that serum levels of this compound correlate strongly with phytanic acid levels and have been investigated for their association with prostate cancer risk. High levels of AMACR expression in prostate tumors suggest a potential link between this compound metabolism and cancer progression .
Research Findings
Recent studies have elucidated several important aspects of this compound's biological activity:
- Oxidation Rates : In healthy controls, the oxidation rates of this compound were significantly higher than those observed in patients with metabolic defects. For instance, control fibroblasts exhibited a wide range of oxidation rates compared to severely reduced rates in patient-derived cells .
- Biomarker Potential : Plasma concentrations of pristanic and phytanic acids are utilized as biomarkers for diagnosing peroxisomal disorders. Elevated levels indicate a disruption in normal fatty acid metabolism .
Data Table: Oxidation Rates of this compound
Patient Type | Oxidation Rate (pmol/h/mg protein) | Control Range (pmol/h/mg protein) |
---|---|---|
Patient 1 | 0.4–1.2 | 75.5–189.9 |
Patient 2 | 9.7–26.6 | 75.5–189.9 |
Patient 3 | 0.2–0.4 | 75.5–189.9 |
Control Group Average | 152.4–489.6 | N/A |
Properties
IUPAC Name |
2,6,10,14-tetramethylpentadecanoic acid | |
---|---|---|
Source | PubChem | |
URL | https://pubchem.ncbi.nlm.nih.gov | |
Description | Data deposited in or computed by PubChem | |
InChI |
InChI=1S/C19H38O2/c1-15(2)9-6-10-16(3)11-7-12-17(4)13-8-14-18(5)19(20)21/h15-18H,6-14H2,1-5H3,(H,20,21) | |
Source | PubChem | |
URL | https://pubchem.ncbi.nlm.nih.gov | |
Description | Data deposited in or computed by PubChem | |
InChI Key |
PAHGJZDQXIOYTH-UHFFFAOYSA-N | |
Source | PubChem | |
URL | https://pubchem.ncbi.nlm.nih.gov | |
Description | Data deposited in or computed by PubChem | |
Canonical SMILES |
CC(C)CCCC(C)CCCC(C)CCCC(C)C(=O)O | |
Source | PubChem | |
URL | https://pubchem.ncbi.nlm.nih.gov | |
Description | Data deposited in or computed by PubChem | |
Molecular Formula |
C19H38O2 | |
Source | PubChem | |
URL | https://pubchem.ncbi.nlm.nih.gov | |
Description | Data deposited in or computed by PubChem | |
DSSTOX Substance ID |
DTXSID00862584 | |
Record name | Pristanic acid | |
Source | EPA DSSTox | |
URL | https://comptox.epa.gov/dashboard/DTXSID00862584 | |
Description | DSSTox provides a high quality public chemistry resource for supporting improved predictive toxicology. | |
Molecular Weight |
298.5 g/mol | |
Source | PubChem | |
URL | https://pubchem.ncbi.nlm.nih.gov | |
Description | Data deposited in or computed by PubChem | |
Physical Description |
Solid | |
Record name | Pristanic acid | |
Source | Human Metabolome Database (HMDB) | |
URL | http://www.hmdb.ca/metabolites/HMDB0000795 | |
Description | The Human Metabolome Database (HMDB) is a freely available electronic database containing detailed information about small molecule metabolites found in the human body. | |
Explanation | HMDB is offered to the public as a freely available resource. Use and re-distribution of the data, in whole or in part, for commercial purposes requires explicit permission of the authors and explicit acknowledgment of the source material (HMDB) and the original publication (see the HMDB citing page). We ask that users who download significant portions of the database cite the HMDB paper in any resulting publications. | |
CAS No. |
1189-37-3 | |
Record name | Pristanic acid | |
Source | CAS Common Chemistry | |
URL | https://commonchemistry.cas.org/detail?cas_rn=1189-37-3 | |
Description | CAS Common Chemistry is an open community resource for accessing chemical information. Nearly 500,000 chemical substances from CAS REGISTRY cover areas of community interest, including common and frequently regulated chemicals, and those relevant to high school and undergraduate chemistry classes. This chemical information, curated by our expert scientists, is provided in alignment with our mission as a division of the American Chemical Society. | |
Explanation | The data from CAS Common Chemistry is provided under a CC-BY-NC 4.0 license, unless otherwise stated. | |
Record name | Pristanic acid | |
Source | ChemIDplus | |
URL | https://pubchem.ncbi.nlm.nih.gov/substance/?source=chemidplus&sourceid=0001189373 | |
Description | ChemIDplus is a free, web search system that provides access to the structure and nomenclature authority files used for the identification of chemical substances cited in National Library of Medicine (NLM) databases, including the TOXNET system. | |
Record name | Pristanic acid | |
Source | EPA DSSTox | |
URL | https://comptox.epa.gov/dashboard/DTXSID00862584 | |
Description | DSSTox provides a high quality public chemistry resource for supporting improved predictive toxicology. | |
Record name | Pristanic acid | |
Source | Human Metabolome Database (HMDB) | |
URL | http://www.hmdb.ca/metabolites/HMDB0000795 | |
Description | The Human Metabolome Database (HMDB) is a freely available electronic database containing detailed information about small molecule metabolites found in the human body. | |
Explanation | HMDB is offered to the public as a freely available resource. Use and re-distribution of the data, in whole or in part, for commercial purposes requires explicit permission of the authors and explicit acknowledgment of the source material (HMDB) and the original publication (see the HMDB citing page). We ask that users who download significant portions of the database cite the HMDB paper in any resulting publications. | |
Retrosynthesis Analysis
AI-Powered Synthesis Planning: Our tool employs the Template_relevance Pistachio, Template_relevance Bkms_metabolic, Template_relevance Pistachio_ringbreaker, Template_relevance Reaxys, Template_relevance Reaxys_biocatalysis model, leveraging a vast database of chemical reactions to predict feasible synthetic routes.
One-Step Synthesis Focus: Specifically designed for one-step synthesis, it provides concise and direct routes for your target compounds, streamlining the synthesis process.
Accurate Predictions: Utilizing the extensive PISTACHIO, BKMS_METABOLIC, PISTACHIO_RINGBREAKER, REAXYS, REAXYS_BIOCATALYSIS database, our tool offers high-accuracy predictions, reflecting the latest in chemical research and data.
Strategy Settings
Precursor scoring | Relevance Heuristic |
---|---|
Min. plausibility | 0.01 |
Model | Template_relevance |
Template Set | Pistachio/Bkms_metabolic/Pistachio_ringbreaker/Reaxys/Reaxys_biocatalysis |
Top-N result to add to graph | 6 |
Feasible Synthetic Routes
Disclaimer and Information on In-Vitro Research Products
Please be aware that all articles and product information presented on BenchChem are intended solely for informational purposes. The products available for purchase on BenchChem are specifically designed for in-vitro studies, which are conducted outside of living organisms. In-vitro studies, derived from the Latin term "in glass," involve experiments performed in controlled laboratory settings using cells or tissues. It is important to note that these products are not categorized as medicines or drugs, and they have not received approval from the FDA for the prevention, treatment, or cure of any medical condition, ailment, or disease. We must emphasize that any form of bodily introduction of these products into humans or animals is strictly prohibited by law. It is essential to adhere to these guidelines to ensure compliance with legal and ethical standards in research and experimentation.