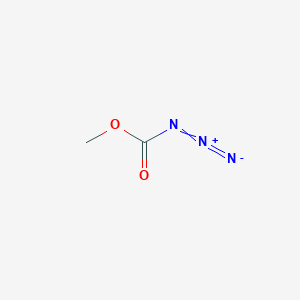
Azido(methoxy)methanone
Overview
Description
Azido(methoxy)methanone is an organic compound with the molecular formula C2H3N3O2 It is characterized by the presence of an azido group (-N3) and a methoxy group (-OCH3) attached to a methanone (carbonyl) group
Mechanism of Action
Target of Action
Azido(methoxy)methanone, like other azido compounds, is primarily used in organic synthesis . The primary targets of azido compounds are typically organic molecules that can undergo reactions with the azido group. The azido group is highly reactive due to the presence of three nitrogen atoms, which can participate in a variety of chemical reactions .
Mode of Action
The mode of action of this compound involves its interaction with other molecules through its azido group. The azido group can undergo a variety of reactions, including nucleophilic addition and cycloaddition reactions . For instance, azido compounds can react with alkynes to form 1,2,3-triazoles in a process known as the Huisgen cycloaddition or “click” reaction . This reaction is often catalyzed by copper(I), leading to the formation of 1,4-disubstituted 1,2,3-triazoles .
Biochemical Pathways
This compound, like other azido compounds, can participate in various biochemical pathways. For instance, azido compounds can be used in the synthesis of various heterocycles . These heterocycles can have various biological activities, depending on their structure and the presence of other functional groups .
Pharmacokinetics
The azido group, for instance, is polar and can form hydrogen bonds, which could influence the compound’s solubility and transport across biological membranes .
Result of Action
The result of the action of this compound would depend on the specific reactions it undergoes. For instance, in the Huisgen cycloaddition reaction, this compound could react with an alkyne to form a 1,2,3-triazole . This reaction could be used to synthesize new compounds with potential biological activity .
Preparation Methods
Synthetic Routes and Reaction Conditions: Azido(methoxy)methanone can be synthesized through several methods, typically involving the introduction of the azido group to a suitable precursor. One common method involves the reaction of methoxyacetyl chloride with sodium azide in an aprotic solvent such as acetonitrile or dimethyl sulfoxide (DMSO). The reaction is typically carried out at low temperatures to prevent decomposition of the azido group.
Industrial Production Methods: Industrial production of this compound may involve similar synthetic routes but on a larger scale. The use of continuous flow reactors can enhance the safety and efficiency of the process, given the potentially hazardous nature of azides. The reaction conditions are carefully controlled to ensure high yield and purity of the product.
Chemical Reactions Analysis
Types of Reactions: Azido(methoxy)methanone undergoes various types of chemical reactions, including:
Substitution Reactions: The azido group can participate in nucleophilic substitution reactions, where it acts as a nucleophile.
Reduction Reactions: The azido group can be reduced to an amine group using reducing agents such as lithium aluminum hydride (LiAlH4) or catalytic hydrogenation.
Cycloaddition Reactions:
Common Reagents and Conditions:
Nucleophilic Substitution: Sodium azide (NaN3) in polar aprotic solvents like acetonitrile or DMSO.
Reduction: Lithium aluminum hydride (LiAlH4) or palladium on carbon (Pd/C) with hydrogen gas.
Cycloaddition: Copper(I) catalysts in the presence of alkynes.
Major Products:
Substitution: Formation of azido-substituted derivatives.
Reduction: Formation of primary amines.
Cycloaddition: Formation of 1,2,3-triazoles.
Scientific Research Applications
Azido(methoxy)methanone has several applications in scientific research:
Chemistry: Used as a precursor in the synthesis of various heterocyclic compounds, including triazoles and other nitrogen-containing heterocycles.
Biology: Employed in bioorthogonal chemistry for labeling and tracking biomolecules.
Industry: Utilized in the production of specialty chemicals and materials, including polymers and explosives.
Comparison with Similar Compounds
Azido(methoxy)methanone can be compared with other azido compounds, such as:
Azidomethane (CH3N3): Similar in structure but lacks the methoxy and carbonyl groups.
Azidoacetone (C3H5N3O): Contains an additional carbon atom and a ketone group.
Azidobenzene (C6H5N3): Contains a benzene ring instead of the methoxy group.
Biological Activity
Azido(methoxy)methanone, a compound characterized by its azido and methoxy functional groups, has garnered attention in various fields of chemical and biological research due to its unique reactivity and potential applications. This article explores the biological activity of this compound, focusing on its mechanisms of action, biochemical pathways, and applications in scientific research.
This compound is an azide compound that exhibits high reactivity due to the presence of the azido group (-N₃). The compound can participate in various chemical reactions, including:
- Nucleophilic Substitution Reactions : The azido group can act as a nucleophile, facilitating the formation of azido-substituted derivatives.
- Reduction Reactions : It can be reduced to primary amines using reducing agents like lithium aluminum hydride (LiAlH₄).
- Cycloaddition Reactions : The compound can undergo cycloaddition reactions, particularly in the presence of alkynes, leading to the formation of 1,2,3-triazoles.
The biological activity of this compound is primarily attributed to its interaction with biomolecules through its reactive azido group. This interaction can lead to:
- Bioorthogonal Chemistry : The azido group allows for selective labeling and tracking of biomolecules in living systems without interfering with native biochemical processes. This property is particularly useful in bioconjugation techniques where specific targeting is required.
- Formation of Nitrene Intermediates : Upon thermal decomposition, azides can generate nitrene intermediates that are highly reactive and can insert into C-H bonds or react with double bonds in organic molecules .
Biochemical Pathways
This compound can participate in various biochemical pathways:
- Synthesis of Heterocycles : The compound serves as a precursor for synthesizing nitrogen-containing heterocycles such as triazoles, which have significant biological activities including antimicrobial and anticancer properties.
- Mechanistic Insights : Studies have shown that substituents on aromatic rings influence the reactivity of azides. For example, electron-donating groups like methoxy can stabilize reactive intermediates, enhancing the overall reaction rates .
Pharmacokinetics
The pharmacokinetic properties of this compound are influenced by its polar azido group, which may affect solubility and membrane permeability. This characteristic is crucial for its application in drug development where effective delivery to target sites is necessary.
Case Studies and Research Findings
Several studies have highlighted the biological implications and applications of this compound:
- Synthesis and Reactivity Studies :
- Bioorthogonal Applications :
- Development of Therapeutic Agents :
Summary of Key Findings
Property/Aspect | Description |
---|---|
Chemical Structure | Contains an azido group (-N₃) and a methoxy group (-OCH₃) |
Reactivity | Engages in nucleophilic substitution, reduction, and cycloaddition reactions |
Biological Applications | Used in bioorthogonal chemistry; precursor for heterocyclic compounds |
Pharmacokinetics | Polar nature influences solubility and transport across membranes |
Case Studies | Demonstrated effectiveness in protein labeling and potential therapeutic uses |
Properties
IUPAC Name |
methyl N-diazocarbamate | |
---|---|---|
Source | PubChem | |
URL | https://pubchem.ncbi.nlm.nih.gov | |
Description | Data deposited in or computed by PubChem | |
InChI |
InChI=1S/C2H3N3O2/c1-7-2(6)4-5-3/h1H3 | |
Source | PubChem | |
URL | https://pubchem.ncbi.nlm.nih.gov | |
Description | Data deposited in or computed by PubChem | |
InChI Key |
QOHNHTHTTUJQEY-UHFFFAOYSA-N | |
Source | PubChem | |
URL | https://pubchem.ncbi.nlm.nih.gov | |
Description | Data deposited in or computed by PubChem | |
Canonical SMILES |
COC(=O)N=[N+]=[N-] | |
Source | PubChem | |
URL | https://pubchem.ncbi.nlm.nih.gov | |
Description | Data deposited in or computed by PubChem | |
Molecular Formula |
C2H3N3O2 | |
Source | PubChem | |
URL | https://pubchem.ncbi.nlm.nih.gov | |
Description | Data deposited in or computed by PubChem | |
DSSTOX Substance ID |
DTXSID30501898 | |
Record name | Methyl carbonazidate | |
Source | EPA DSSTox | |
URL | https://comptox.epa.gov/dashboard/DTXSID30501898 | |
Description | DSSTox provides a high quality public chemistry resource for supporting improved predictive toxicology. | |
Molecular Weight |
101.06 g/mol | |
Source | PubChem | |
URL | https://pubchem.ncbi.nlm.nih.gov | |
Description | Data deposited in or computed by PubChem | |
CAS No. |
1516-56-9 | |
Record name | Methyl carbonazidate | |
Source | EPA DSSTox | |
URL | https://comptox.epa.gov/dashboard/DTXSID30501898 | |
Description | DSSTox provides a high quality public chemistry resource for supporting improved predictive toxicology. | |
Disclaimer and Information on In-Vitro Research Products
Please be aware that all articles and product information presented on BenchChem are intended solely for informational purposes. The products available for purchase on BenchChem are specifically designed for in-vitro studies, which are conducted outside of living organisms. In-vitro studies, derived from the Latin term "in glass," involve experiments performed in controlled laboratory settings using cells or tissues. It is important to note that these products are not categorized as medicines or drugs, and they have not received approval from the FDA for the prevention, treatment, or cure of any medical condition, ailment, or disease. We must emphasize that any form of bodily introduction of these products into humans or animals is strictly prohibited by law. It is essential to adhere to these guidelines to ensure compliance with legal and ethical standards in research and experimentation.