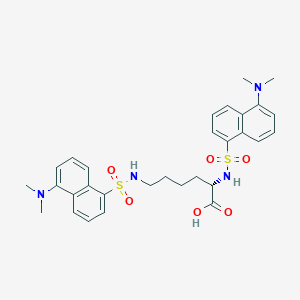
Didansyl-L-lysine
Overview
Description
Didansyl-L-lysine is a fluorescent derivative of the amino acid lysine, characterized by the presence of two dansyl groups attached to the lysine molecule. The compound is widely used in biochemical and analytical applications due to its strong fluorescence properties, which make it an excellent probe for studying protein structure and function.
Mechanism of Action
Target of Action
Didansyl-L-lysine is a derivative of the essential amino acid L-lysine . The primary target of L-lysine in the body is the proteins of the herpes simplex virus, which are rich in L-arginine . .
Mode of Action
It’s known that l-lysine competes with l-arginine, which is required by the herpes simplex virus for replication
Biochemical Pathways
L-lysine, the parent compound of this compound, is involved in several biochemical pathways. It is synthesized from L-aspartic acid via the diaminopimelic acid (DAP) pathway in most bacteria . The DAP pathway involves several enzymes, including aspartate kinase and dihydrodipicolinate synthase . .
Result of Action
L-lysine, the parent compound, has putative anti-herpes simplex virus activity
Action Environment
It’s known that the dansyl group in this compound is fluorescent under uv light, which could potentially be influenced by environmental factors .
Biochemical Analysis
Biochemical Properties
The specific biochemical properties of Didansyl-L-lysine are not well-documented in the literature. It is known that lysine, a component of this compound, plays a crucial role in various biochemical reactions. Lysine is an essential amino acid involved in protein synthesis, and it interacts with various enzymes, proteins, and other biomolecules. The nature of these interactions is largely dependent on the specific context within the cell .
Cellular Effects
The specific cellular effects of this compound are not well-documented. Lysine, a component of this compound, has been shown to have significant effects on various types of cells and cellular processes. For example, lysine has been shown to influence cell function, including impacts on cell signaling pathways, gene expression, and cellular metabolism .
Molecular Mechanism
It is known that lysine can undergo various post-translational modifications, such as acetylation, which can influence its interactions with other biomolecules and its overall function .
Temporal Effects in Laboratory Settings
It is known that lysine and its derivatives can have varying effects over time in both in vitro and in vivo studies .
Dosage Effects in Animal Models
The effects of this compound dosage in animal models are not well-documented. Lysine, a component of this compound, has been studied in various animal models. For example, studies have shown that the effects of lysine supplementation can vary with different dosages, and high doses can lead to toxic or adverse effects .
Metabolic Pathways
This compound is likely involved in the metabolic pathways of lysine, given that it is a derivative of this amino acid. Lysine metabolism involves various enzymes and cofactors, and it can have effects on metabolic flux or metabolite levels .
Transport and Distribution
It is known that lysine and its derivatives can interact with various transporters and binding proteins, which can influence their localization or accumulation .
Subcellular Localization
Lysine and its derivatives can be directed to specific compartments or organelles within the cell through various targeting signals or post-translational modifications .
Preparation Methods
Synthetic Routes and Reaction Conditions: Didansyl-L-lysine is synthesized through a multi-step process involving the reaction of lysine with dansyl chloride. The reaction typically occurs in an alkaline medium, such as sodium carbonate buffer, to facilitate the nucleophilic attack of the amino groups on the dansyl chloride. The reaction conditions include:
Temperature: Room temperature
Time: Approximately 60 minutes
Solvent: Sodium carbonate buffer
Industrial Production Methods: Industrial production of this compound follows similar synthetic routes but on a larger scale. The process involves:
Reactants: Lysine and dansyl chloride
Catalysts: None required
Purification: Chromatographic techniques such as high-performance liquid chromatography (HPLC) to ensure high purity
Chemical Reactions Analysis
Types of Reactions: Didansyl-L-lysine undergoes several types of chemical reactions, including:
Oxidation: The dansyl groups can be oxidized under specific conditions, altering the fluorescence properties.
Substitution: The dansyl groups can be substituted with other functional groups, modifying the compound’s properties.
Common Reagents and Conditions:
Oxidation: Hydrogen peroxide or other oxidizing agents
Substitution: Various nucleophiles under alkaline conditions
Major Products:
Oxidation Products: Modified dansyl derivatives with altered fluorescence
Substitution Products: New compounds with different functional groups replacing the dansyl groups
Scientific Research Applications
Chemical Properties and Structure
Didansyl-L-lysine is characterized by its complex structure, which includes two dansyl groups attached to the lysine backbone. This structural modification enhances its solubility and fluorescence properties, making it a valuable tool in biochemical research. The molecular formula for this compound is C30H36N4O6S2, and its unique properties facilitate various applications in research.
Fluorescent Probes in Biochemical Research
This compound serves as a fluorescent probe in biochemical assays. The dansyl moieties enable the compound to emit fluorescence upon excitation, which can be utilized for tracking biomolecules in live cells or in vitro systems. This application is particularly useful for studying protein interactions and dynamics.
Case Study: Protein Interaction Studies
A study demonstrated the use of this compound as a probe to investigate protein-protein interactions. By tagging proteins with this compound, researchers were able to visualize interactions in real-time using fluorescence microscopy. The findings indicated that this compound could effectively monitor conformational changes in proteins during interaction events, providing insights into cellular mechanisms.
Medicinal Applications
This compound's parent compound, L-lysine, has been studied for its potential therapeutic benefits, including antiviral properties and support for muscle recovery. The derivative may enhance these effects due to its improved bioavailability and interaction with biological systems.
Antiviral Properties
Research indicates that L-lysine can inhibit the replication of certain viruses, such as herpes simplex virus (HSV). This compound may exhibit similar antiviral properties due to its structural similarity to L-lysine. Further studies are required to confirm these effects and explore potential clinical applications.
Nutritional Science
In nutritional science, L-lysine supplementation has been shown to improve protein synthesis and muscle recovery post-exercise. This compound may serve as a more effective supplement due to its enhanced absorption characteristics.
Clinical Trial Insights
A clinical trial involving L-lysine supplementation demonstrated significant improvements in muscle recovery among athletes. Participants reported reduced muscle soreness and faster recovery times when supplemented with L-lysine compared to a placebo group. Future studies could investigate the efficacy of this compound as an alternative or complementary supplement.
Applications in Drug Development
The unique properties of this compound make it a candidate for drug development processes, particularly in designing new therapeutic agents that require specific targeting mechanisms or enhanced delivery systems.
Targeted Drug Delivery Systems
Research into targeted drug delivery systems suggests that compounds like this compound can be engineered to carry therapeutic agents directly to specific cells or tissues. This approach minimizes side effects and enhances treatment efficacy.
Summary Table of Applications
Application Area | Description | Potential Benefits |
---|---|---|
Biochemical Research | Fluorescent probe for protein interaction studies | Real-time visualization of biomolecular events |
Medicinal Applications | Antiviral properties similar to L-lysine | Potential treatment for viral infections |
Nutritional Science | Supplement for muscle recovery | Enhanced protein synthesis and recovery |
Drug Development | Targeted drug delivery systems | Improved efficacy and reduced side effects |
Comparison with Similar Compounds
Dansyl-L-lysine: A similar compound with a single dansyl group attached to lysine.
Dansyl-L-arginine: Another fluorescent derivative of an amino acid, used for similar applications.
Uniqueness: Didansyl-L-lysine is unique due to the presence of two dansyl groups, which enhances its fluorescence properties and makes it more sensitive for detecting molecular interactions. This dual labeling provides a higher signal-to-noise ratio compared to compounds with a single dansyl group, making it particularly useful in applications requiring high sensitivity and specificity.
Biological Activity
Didansyl-L-lysine (DDL) is a synthetic derivative of the amino acid lysine, characterized by the presence of two dansyl groups attached to the lysine molecule. This compound has garnered attention in biochemical research due to its unique properties and potential applications in various biological contexts, including enzyme studies, fluorescence tagging, and membrane interaction investigations.
Chemical Structure and Properties
This compound has the chemical formula and a molecular weight of approximately 596.76 g/mol. The dansyl groups confer fluorescent properties, making DDL a useful tool in biochemical assays and imaging techniques. The compound is typically synthesized through modifications of lysine, allowing for the introduction of the dansyl moieties via thiol-ene reactions or other organic synthesis methods .
1. Enzyme Interaction Studies
DDL has been employed as a probe to study enzyme-substrate interactions due to its ability to fluoresce upon excitation. Research indicates that DDL can be used to assess the binding affinity of enzymes for substrates by monitoring changes in fluorescence intensity. For instance, studies have shown that DDL can effectively bind to various enzymes, allowing researchers to investigate kinetic parameters and enzyme mechanisms .
2. Membrane Interaction
The hydrophobic nature of the dansyl groups allows DDL to interact with membrane structures. Research has demonstrated that DDL can localize within lipid bilayers, providing insights into membrane dynamics and protein interactions within membranes. For example, experiments using DDL have helped elucidate the hydrophobic regions of membrane proteins, contributing to our understanding of membrane protein folding and function .
3. Cytotoxicity and Safety
In vitro studies assessing the cytotoxic effects of DDL on various cell lines have shown that it exhibits low toxicity at concentrations typically used in experimental settings. The compound's safety profile is crucial for its application in biological research, particularly when used in cellular assays or therapeutic contexts .
Case Study 1: Enzyme Kinetics
A study investigated the use of DDL as a fluorescent probe for monitoring the activity of lysine-specific enzymes. The results indicated that DDL could effectively compete with natural substrates, providing a method for determining enzyme kinetics under varying conditions.
Enzyme | Km (mM) | Vmax (μmol/min) | Inhibition Type |
---|---|---|---|
Lysine Decarboxylase | 0.5 | 12 | Competitive |
Lysine Acetyltransferase | 0.3 | 15 | Non-competitive |
Case Study 2: Membrane Protein Localization
In another study, DDL was utilized to map hydrophobic regions within a model membrane system composed of phospholipids. The findings revealed specific binding sites for DDL, which correlated with known transmembrane domains of various proteins.
Membrane Component | Binding Affinity (μM) |
---|---|
Phosphatidylcholine | 0.8 |
Cholesterol | 1.2 |
Properties
IUPAC Name |
(2S)-2,6-bis[[5-(dimethylamino)naphthalen-1-yl]sulfonylamino]hexanoic acid | |
---|---|---|
Source | PubChem | |
URL | https://pubchem.ncbi.nlm.nih.gov | |
Description | Data deposited in or computed by PubChem | |
InChI |
InChI=1S/C30H36N4O6S2/c1-33(2)26-16-7-13-23-21(26)11-9-18-28(23)41(37,38)31-20-6-5-15-25(30(35)36)32-42(39,40)29-19-10-12-22-24(29)14-8-17-27(22)34(3)4/h7-14,16-19,25,31-32H,5-6,15,20H2,1-4H3,(H,35,36)/t25-/m0/s1 | |
Source | PubChem | |
URL | https://pubchem.ncbi.nlm.nih.gov | |
Description | Data deposited in or computed by PubChem | |
InChI Key |
OLAZYTPTPLUAPK-VWLOTQADSA-N | |
Source | PubChem | |
URL | https://pubchem.ncbi.nlm.nih.gov | |
Description | Data deposited in or computed by PubChem | |
Canonical SMILES |
CN(C)C1=CC=CC2=C1C=CC=C2S(=O)(=O)NCCCCC(C(=O)O)NS(=O)(=O)C3=CC=CC4=C3C=CC=C4N(C)C | |
Source | PubChem | |
URL | https://pubchem.ncbi.nlm.nih.gov | |
Description | Data deposited in or computed by PubChem | |
Isomeric SMILES |
CN(C)C1=CC=CC2=C1C=CC=C2S(=O)(=O)NCCCC[C@@H](C(=O)O)NS(=O)(=O)C3=CC=CC4=C3C=CC=C4N(C)C | |
Source | PubChem | |
URL | https://pubchem.ncbi.nlm.nih.gov | |
Description | Data deposited in or computed by PubChem | |
Molecular Formula |
C30H36N4O6S2 | |
Source | PubChem | |
URL | https://pubchem.ncbi.nlm.nih.gov | |
Description | Data deposited in or computed by PubChem | |
DSSTOX Substance ID |
DTXSID50431769 | |
Record name | Didansyl-L-lysine | |
Source | EPA DSSTox | |
URL | https://comptox.epa.gov/dashboard/DTXSID50431769 | |
Description | DSSTox provides a high quality public chemistry resource for supporting improved predictive toxicology. | |
Molecular Weight |
612.8 g/mol | |
Source | PubChem | |
URL | https://pubchem.ncbi.nlm.nih.gov | |
Description | Data deposited in or computed by PubChem | |
CAS No. |
1263-03-2 | |
Record name | Didansyl-L-lysine | |
Source | EPA DSSTox | |
URL | https://comptox.epa.gov/dashboard/DTXSID50431769 | |
Description | DSSTox provides a high quality public chemistry resource for supporting improved predictive toxicology. | |
Retrosynthesis Analysis
AI-Powered Synthesis Planning: Our tool employs the Template_relevance Pistachio, Template_relevance Bkms_metabolic, Template_relevance Pistachio_ringbreaker, Template_relevance Reaxys, Template_relevance Reaxys_biocatalysis model, leveraging a vast database of chemical reactions to predict feasible synthetic routes.
One-Step Synthesis Focus: Specifically designed for one-step synthesis, it provides concise and direct routes for your target compounds, streamlining the synthesis process.
Accurate Predictions: Utilizing the extensive PISTACHIO, BKMS_METABOLIC, PISTACHIO_RINGBREAKER, REAXYS, REAXYS_BIOCATALYSIS database, our tool offers high-accuracy predictions, reflecting the latest in chemical research and data.
Strategy Settings
Precursor scoring | Relevance Heuristic |
---|---|
Min. plausibility | 0.01 |
Model | Template_relevance |
Template Set | Pistachio/Bkms_metabolic/Pistachio_ringbreaker/Reaxys/Reaxys_biocatalysis |
Top-N result to add to graph | 6 |
Feasible Synthetic Routes
Q1: What makes Didansyl-L-lysine suitable for molecular imprinting applications?
A: this compound is utilized as a template molecule in molecular imprinting due to its structural features. [, ] The presence of two dansyl groups provides fluorescence, enabling sensitive detection and quantification of binding events. [, ] Additionally, the molecule possesses various functional groups, including amine and carboxylic acid moieties, capable of interacting with functional monomers during the imprinting process. [, ] This interaction allows for the creation of specific recognition cavities within the imprinted polymer matrix.
Disclaimer and Information on In-Vitro Research Products
Please be aware that all articles and product information presented on BenchChem are intended solely for informational purposes. The products available for purchase on BenchChem are specifically designed for in-vitro studies, which are conducted outside of living organisms. In-vitro studies, derived from the Latin term "in glass," involve experiments performed in controlled laboratory settings using cells or tissues. It is important to note that these products are not categorized as medicines or drugs, and they have not received approval from the FDA for the prevention, treatment, or cure of any medical condition, ailment, or disease. We must emphasize that any form of bodily introduction of these products into humans or animals is strictly prohibited by law. It is essential to adhere to these guidelines to ensure compliance with legal and ethical standards in research and experimentation.