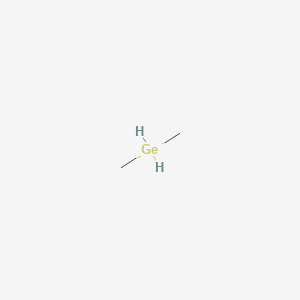
Germane, dimethyl-
- Click on QUICK INQUIRY to receive a quote from our team of experts.
- With the quality product at a COMPETITIVE price, you can focus more on your research.
Overview
Description
Germane, dimethyl- is an organogermanium compound with the chemical formula ( \text{C}_2\text{H}_8\text{Ge} ). It is a colorless gas at room temperature and is known for its applications in various fields, including materials science and organic synthesis. This compound is part of the broader class of organometallic compounds, which are characterized by the presence of a metal-carbon bond.
Preparation Methods
Synthetic Routes and Reaction Conditions: Germane, dimethyl- can be synthesized through several methods. One common approach involves the reaction of germanium tetrachloride with methyl lithium or methyl magnesium bromide. The reaction proceeds as follows: [ \text{GeCl}_4 + 2 \text{CH}_3\text{Li} \rightarrow \text{Ge}(\text{CH}_3)_2\text{Cl}_2 + 2 \text{LiCl} ] [ \text{Ge}(\text{CH}_3)_2\text{Cl}_2 + 2 \text{CH}_3\text{Li} \rightarrow \text{Ge}(\text{CH}_3)_4 + 2 \text{LiCl} ]
Industrial Production Methods: In industrial settings, germane, dimethyl- is often produced through the reduction of germanium dioxide with hydrogen in the presence of a catalyst. This method is advantageous due to its scalability and cost-effectiveness.
Chemical Reactions Analysis
Types of Reactions: Germane, dimethyl- undergoes various chemical reactions, including:
Oxidation: When exposed to oxygen, it can form germanium dioxide and water. [ \text{Ge}(\text{CH}_3)_2 + 2 \text{O}_2 \rightarrow \text{GeO}_2 + 2 \text{H}_2\text{O} ]
Reduction: It can be reduced to elemental germanium under specific conditions.
Substitution: Germane, dimethyl- can participate in substitution reactions with halogens to form halogermanes.
Common Reagents and Conditions:
Oxidation: Oxygen or other oxidizing agents.
Reduction: Hydrogen gas in the presence of a catalyst.
Substitution: Halogens such as chlorine or bromine.
Major Products:
Oxidation: Germanium dioxide and water.
Reduction: Elemental germanium.
Substitution: Halogermanes.
Scientific Research Applications
Germane, dimethyl- has several applications in scientific research:
Chemistry: It is used as a precursor in the synthesis of other organogermanium compounds.
Materials Science: It is utilized in the production of germanium-containing semiconductors and thin films.
Biology and Medicine: Research is ongoing into its potential use in pharmaceuticals and as a therapeutic agent.
Industry: It is employed in the manufacturing of electronic components and as a catalyst in various chemical reactions.
Mechanism of Action
The mechanism of action of germane, dimethyl- involves its interaction with various molecular targets. In catalytic applications, it acts by facilitating the activation of small molecules through its metal center. The pathways involved often include the formation of intermediate complexes that enhance the reactivity of the substrate.
Comparison with Similar Compounds
- Silane, dimethyl-
- Stannane, dimethyl-
- Plumbane, dimethyl-
Comparison: Germane, dimethyl- is unique among its group 14 analogs due to its specific electronic properties and reactivity. While silane, dimethyl- and stannane, dimethyl- share similar structural features, germane, dimethyl- exhibits distinct reactivity patterns, particularly in its ability to form stable complexes with various ligands. This makes it particularly valuable in applications requiring precise control over reactivity and selectivity.
Properties
IUPAC Name |
dimethylgermane |
Source
|
---|---|---|
Source | PubChem | |
URL | https://pubchem.ncbi.nlm.nih.gov | |
Description | Data deposited in or computed by PubChem | |
InChI |
InChI=1S/C2H8Ge/c1-3-2/h3H2,1-2H3 |
Source
|
Source | PubChem | |
URL | https://pubchem.ncbi.nlm.nih.gov | |
Description | Data deposited in or computed by PubChem | |
InChI Key |
RUIGDFHKELAHJL-UHFFFAOYSA-N |
Source
|
Source | PubChem | |
URL | https://pubchem.ncbi.nlm.nih.gov | |
Description | Data deposited in or computed by PubChem | |
Canonical SMILES |
C[GeH2]C |
Source
|
Source | PubChem | |
URL | https://pubchem.ncbi.nlm.nih.gov | |
Description | Data deposited in or computed by PubChem | |
Molecular Formula |
C2H8Ge |
Source
|
Source | PubChem | |
URL | https://pubchem.ncbi.nlm.nih.gov | |
Description | Data deposited in or computed by PubChem | |
Molecular Weight |
104.72 g/mol |
Source
|
Source | PubChem | |
URL | https://pubchem.ncbi.nlm.nih.gov | |
Description | Data deposited in or computed by PubChem | |
CAS No. |
1449-64-5 |
Source
|
Record name | Germane, dimethyl- | |
Source | ChemIDplus | |
URL | https://pubchem.ncbi.nlm.nih.gov/substance/?source=chemidplus&sourceid=0001449645 | |
Description | ChemIDplus is a free, web search system that provides access to the structure and nomenclature authority files used for the identification of chemical substances cited in National Library of Medicine (NLM) databases, including the TOXNET system. | |
Disclaimer and Information on In-Vitro Research Products
Please be aware that all articles and product information presented on BenchChem are intended solely for informational purposes. The products available for purchase on BenchChem are specifically designed for in-vitro studies, which are conducted outside of living organisms. In-vitro studies, derived from the Latin term "in glass," involve experiments performed in controlled laboratory settings using cells or tissues. It is important to note that these products are not categorized as medicines or drugs, and they have not received approval from the FDA for the prevention, treatment, or cure of any medical condition, ailment, or disease. We must emphasize that any form of bodily introduction of these products into humans or animals is strictly prohibited by law. It is essential to adhere to these guidelines to ensure compliance with legal and ethical standards in research and experimentation.