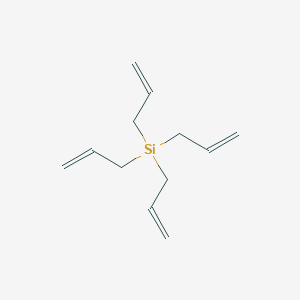
Tetraallylsilane
Overview
Description
Tetraallylsilane is an organosilicon compound with the molecular formula C12H20Si. It is a colorless to light yellow liquid that is insoluble in water. This compound is a special silicone monomer characterized by its stable Si-C bonds, which have a bond dissociation energy similar to that of C-C bonds. This stability makes it a valuable raw material in the preparation of silicone rubber and other industrial applications .
Synthetic Routes and Reaction Conditions:
-
Method 1:
Reactants: Allyl magnesium bromide (Allyl MgBr) and silicon tetrachloride (SiCl4).
Procedure: Allyl magnesium bromide in tetrahydrofuran (THF) is added dropwise to a solution of silicon tetrachloride in THF at room temperature. The reaction mixture is stirred for 2 hours at room temperature and then refluxed overnight. After completion, the mixture is cooled, poured into ice water, and extracted with ether.
-
Method 2:
Reactants: 3-bromopropene, magnesium powder, and silicon tetrachloride.
Procedure: Magnesium powder is activated with iodine and then reacted with 3-bromopropene in a mixture of toluene and tetrahydrofuran. Silicon tetrachloride dissolved in tetrahydrofuran is added dropwise to the reaction mixture.
Industrial Production Methods: Industrial production of this compound typically follows similar synthetic routes but on a larger scale, with optimized reaction conditions to ensure high yield and purity. The use of continuous flow reactors and advanced purification techniques like distillation under reduced pressure are common in industrial settings.
Types of Reactions:
-
Rearrangement Reactions:
- This compound can undergo mono or double rearrangement when treated with iodine. The extent of rearrangement depends on the equivalents of iodine used. One equivalent of iodine results in high yields of mono-rearranged products, while excess iodine causes double rearrangement .
-
Substitution Reactions:
- This compound can participate in substitution reactions where the allyl groups are replaced by other functional groups under appropriate conditions.
Common Reagents and Conditions:
Iodine (I2): Used in rearrangement reactions to produce mono or double-rearranged products.
Grignard Reagents: Used in the synthesis of this compound and in substitution reactions.
Major Products:
Mono-rearranged Products: Formed when this compound is treated with one equivalent of iodine.
Double-rearranged Products: Formed when this compound is treated with excess iodine.
Mechanism of Action
Target of Action
Tetraallylsilane is a type of allyl silane . Allyl silanes are special silicone monomers that have a Si-C bond . This bond is reactive, similar to the C-C bond, and has very low polarity . The primary targets of this compound are therefore likely to be molecules or structures that can react with the Si-C bond.
Pharmacokinetics
It is known that the compound has a molecular weight of 19237 , which could influence its bioavailability
Scientific Research Applications
Tetraallylsilane has several applications in scientific research, including:
Comparison with Similar Compounds
Diallylsilane: Contains two allyl groups attached to silicon.
Triethylsilane: Contains three ethyl groups attached to silicon and is used as a reducing agent in organic synthesis.
Tetrasilane: A silane with the structure formula SiH3-(SiH2)2-SiH3, analogous to butane.
Uniqueness of Tetraallylsilane: this compound is unique due to its four allyl groups attached to a single silicon atom, providing multiple sites for chemical reactions. This makes it a versatile compound in synthetic chemistry, particularly in the formation of silicon-stereogenic compounds and silacycles .
Biological Activity
Tetraallylsilane (TAS) is a silane compound with significant potential in various biological applications, particularly in drug delivery and antimicrobial activity. This article explores the biological activity of TAS, highlighting its mechanisms, effectiveness, and relevant case studies.
Chemical Structure and Properties
This compound is characterized by its silicon atom bonded to four allyl groups. The structure can be represented as:
This configuration allows TAS to participate in various chemical reactions, including those that enhance its biological activity.
Mechanisms of Biological Activity
- Cell Penetration : TAS has shown the ability to penetrate cellular membranes effectively. This property is crucial for its use as a carrier for therapeutic agents, particularly in delivering RNA interference (RNAi) molecules across the blood-brain barrier .
- Antimicrobial Properties : Studies indicate that compounds related to TAS exhibit antimicrobial activity. The mechanism involves disrupting bacterial membranes through electrostatic interactions, which is particularly effective against Gram-negative bacteria .
- Gene Delivery : TAS derivatives have been utilized in dendrimer-based systems for gene delivery. These systems leverage the silane's ability to form stable complexes with nucleic acids, enhancing cellular uptake and therapeutic efficacy .
Antimicrobial Activity
A study evaluated the antibacterial effectiveness of dendritic structures derived from TAS against various pathogens, including Escherichia coli and Pseudomonas aeruginosa. The results demonstrated a significant reduction in bacterial viability, with effectiveness attributed to membrane disruption caused by the positively charged dendrimers .
Pathogen | Dendrimer Type | Activity |
---|---|---|
E. coli | Polycationic Dendrimers | 80% reduction in viability |
P. aeruginosa | Peptido-Dendrimers | 75% reduction in growth |
Gene Delivery Efficiency
In a gene therapy context, TAS-based dendrimers were tested for their ability to deliver siRNA targeting cancer cells. The study found that these dendrimers could achieve over 70% knockdown of target genes in vitro, demonstrating their potential as effective gene delivery vehicles .
Target Gene | Cell Type | Knockdown Efficiency |
---|---|---|
Hsp27 | Prostate Cancer Cells (PC-3) | 80% |
p24 | HIV-infected Lymphocytes | 60% |
Safety and Toxicity Profile
While TAS exhibits promising biological activities, its safety profile is crucial for clinical applications. Studies suggest that carbosilane dendrimers based on TAS are significantly less toxic than other classes of dendrimers, making them suitable candidates for therapeutic use .
Properties
IUPAC Name |
tetrakis(prop-2-enyl)silane | |
---|---|---|
Source | PubChem | |
URL | https://pubchem.ncbi.nlm.nih.gov | |
Description | Data deposited in or computed by PubChem | |
InChI |
InChI=1S/C12H20Si/c1-5-9-13(10-6-2,11-7-3)12-8-4/h5-8H,1-4,9-12H2 | |
Source | PubChem | |
URL | https://pubchem.ncbi.nlm.nih.gov | |
Description | Data deposited in or computed by PubChem | |
InChI Key |
AKRQMTFHUVDMIL-UHFFFAOYSA-N | |
Source | PubChem | |
URL | https://pubchem.ncbi.nlm.nih.gov | |
Description | Data deposited in or computed by PubChem | |
Canonical SMILES |
C=CC[Si](CC=C)(CC=C)CC=C | |
Source | PubChem | |
URL | https://pubchem.ncbi.nlm.nih.gov | |
Description | Data deposited in or computed by PubChem | |
Molecular Formula |
C12H20Si | |
Source | PubChem | |
URL | https://pubchem.ncbi.nlm.nih.gov | |
Description | Data deposited in or computed by PubChem | |
DSSTOX Substance ID |
DTXSID80340557 | |
Record name | Tetraallylsilane | |
Source | EPA DSSTox | |
URL | https://comptox.epa.gov/dashboard/DTXSID80340557 | |
Description | DSSTox provides a high quality public chemistry resource for supporting improved predictive toxicology. | |
Molecular Weight |
192.37 g/mol | |
Source | PubChem | |
URL | https://pubchem.ncbi.nlm.nih.gov | |
Description | Data deposited in or computed by PubChem | |
CAS No. |
1112-66-9 | |
Record name | Tetraallylsilane | |
Source | EPA DSSTox | |
URL | https://comptox.epa.gov/dashboard/DTXSID80340557 | |
Description | DSSTox provides a high quality public chemistry resource for supporting improved predictive toxicology. | |
Record name | 1112-66-9 | |
Source | European Chemicals Agency (ECHA) | |
URL | https://echa.europa.eu/information-on-chemicals | |
Description | The European Chemicals Agency (ECHA) is an agency of the European Union which is the driving force among regulatory authorities in implementing the EU's groundbreaking chemicals legislation for the benefit of human health and the environment as well as for innovation and competitiveness. | |
Explanation | Use of the information, documents and data from the ECHA website is subject to the terms and conditions of this Legal Notice, and subject to other binding limitations provided for under applicable law, the information, documents and data made available on the ECHA website may be reproduced, distributed and/or used, totally or in part, for non-commercial purposes provided that ECHA is acknowledged as the source: "Source: European Chemicals Agency, http://echa.europa.eu/". Such acknowledgement must be included in each copy of the material. ECHA permits and encourages organisations and individuals to create links to the ECHA website under the following cumulative conditions: Links can only be made to webpages that provide a link to the Legal Notice page. | |
Retrosynthesis Analysis
AI-Powered Synthesis Planning: Our tool employs the Template_relevance Pistachio, Template_relevance Bkms_metabolic, Template_relevance Pistachio_ringbreaker, Template_relevance Reaxys, Template_relevance Reaxys_biocatalysis model, leveraging a vast database of chemical reactions to predict feasible synthetic routes.
One-Step Synthesis Focus: Specifically designed for one-step synthesis, it provides concise and direct routes for your target compounds, streamlining the synthesis process.
Accurate Predictions: Utilizing the extensive PISTACHIO, BKMS_METABOLIC, PISTACHIO_RINGBREAKER, REAXYS, REAXYS_BIOCATALYSIS database, our tool offers high-accuracy predictions, reflecting the latest in chemical research and data.
Strategy Settings
Precursor scoring | Relevance Heuristic |
---|---|
Min. plausibility | 0.01 |
Model | Template_relevance |
Template Set | Pistachio/Bkms_metabolic/Pistachio_ringbreaker/Reaxys/Reaxys_biocatalysis |
Top-N result to add to graph | 6 |
Feasible Synthetic Routes
Disclaimer and Information on In-Vitro Research Products
Please be aware that all articles and product information presented on BenchChem are intended solely for informational purposes. The products available for purchase on BenchChem are specifically designed for in-vitro studies, which are conducted outside of living organisms. In-vitro studies, derived from the Latin term "in glass," involve experiments performed in controlled laboratory settings using cells or tissues. It is important to note that these products are not categorized as medicines or drugs, and they have not received approval from the FDA for the prevention, treatment, or cure of any medical condition, ailment, or disease. We must emphasize that any form of bodily introduction of these products into humans or animals is strictly prohibited by law. It is essential to adhere to these guidelines to ensure compliance with legal and ethical standards in research and experimentation.