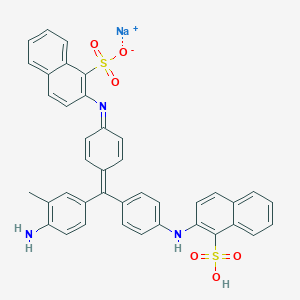
C.I. Direct Blue 41
Overview
Description
C.I. Direct Blue 41 is a synthetic dye belonging to the class of direct dyes, which are anionic dyes with substantivity for cellulosic fibers. These dyes are typically applied from an aqueous dyebath containing an electrolyte, such as sodium chloride or sodium sulfate. This compound is widely used in the textile industry for dyeing cotton, silk, and viscose fibers due to its ability to produce bright shades. it exhibits poor wash fastness, which necessitates aftertreatments to improve its durability .
Preparation Methods
Synthetic Routes and Reaction Conditions
The synthesis of C.I. Direct Blue 41 involves the coupling of diazotized aromatic amines with aromatic coupling components. The reaction typically takes place in an aqueous medium under controlled pH and temperature conditions. The diazotization process involves the reaction of an aromatic amine with nitrous acid, generated in situ from sodium nitrite and hydrochloric acid. The resulting diazonium salt is then coupled with an aromatic compound containing electron-donating groups, such as hydroxyl or amino groups, to form the azo dye.
Industrial Production Methods
Industrial production of this compound follows a similar synthetic route but on a larger scale. The process involves the use of large reactors and precise control of reaction parameters to ensure consistent dye quality. The dye is then isolated, purified, and standardized to meet industry specifications. The final product is typically supplied as a powder or granules, which are easily soluble in water for application in dyeing processes .
Chemical Reactions Analysis
Types of Reactions
C.I. Direct Blue 41 undergoes various chemical reactions, including:
Oxidation: The dye can be oxidized under specific conditions, leading to the breakdown of the azo bonds and the formation of smaller aromatic compounds.
Reduction: Reduction reactions can cleave the azo bonds, resulting in the formation of aromatic amines.
Substitution: The dye can undergo substitution reactions, particularly at the aromatic rings, where substituents such as halogens or alkyl groups can replace hydrogen atoms.
Common Reagents and Conditions
Oxidation: Common oxidizing agents include hydrogen peroxide and potassium permanganate. The reactions are typically carried out in acidic or neutral conditions.
Reduction: Reducing agents such as sodium dithionite or zinc dust in acidic conditions are commonly used.
Substitution: Halogenation reactions often use reagents like chlorine or bromine in the presence of a catalyst.
Major Products Formed
Oxidation: Smaller aromatic compounds, such as phenols and quinones.
Reduction: Aromatic amines, which can be further processed or used as intermediates in other chemical syntheses.
Substitution: Halogenated or alkylated derivatives of the original dye
Scientific Research Applications
C.I. Direct Blue 41 has a wide range of applications in scientific research, including:
Chemistry: Used as a pH indicator and in various analytical techniques, such as high-performance liquid chromatography (HPLC) for the separation and analysis of complex mixtures.
Biology: Employed as a biological stain for visualizing cellular components under a microscope.
Medicine: Investigated for its potential use in diagnostic assays and as a therapeutic agent in certain medical conditions.
Industry: Extensively used in the textile industry for dyeing fabrics, as well as in the paper and leather industries for coloring products
Mechanism of Action
The mechanism of action of C.I. Direct Blue 41 involves its interaction with the target substrate, typically cellulosic fibers. The dye molecules adhere to the fibers through weak hydrogen bonding and Van der Waals forces. The flat shape and length of the dye molecules enable them to align alongside the cellulose fibers, maximizing these interactions. The dye does not form permanent chemical bonds with the fibers, which accounts for its poor wash fastness. In biological applications, the dye interacts with cellular components, allowing for visualization under a microscope .
Comparison with Similar Compounds
C.I. Direct Blue 41 can be compared with other direct dyes, such as:
C.I. Direct Blue 1: Similar in structure and application but may differ in shade and fastness properties.
C.I. Direct Orange 25: Another direct dye with different chromophore and color properties.
C.I. Direct Red 81: Used for dyeing cellulosic fibers but produces a red shade instead of blue.
Uniqueness
This compound is unique in its specific shade of blue and its application in various industries. Its ability to produce bright shades on cellulosic fibers makes it a valuable dye in the textile industry. its poor wash fastness requires aftertreatments to enhance its durability .
Properties
IUPAC Name |
sodium;2-[[4-[(4-amino-3-methylphenyl)-[4-[(1-sulfonaphthalen-2-yl)amino]phenyl]methylidene]cyclohexa-2,5-dien-1-ylidene]amino]naphthalene-1-sulfonate | |
---|---|---|
Source | PubChem | |
URL | https://pubchem.ncbi.nlm.nih.gov | |
Description | Data deposited in or computed by PubChem | |
InChI |
InChI=1S/C40H31N3O6S2.Na/c1-25-24-30(14-21-35(25)41)38(28-10-17-31(18-11-28)42-36-22-15-26-6-2-4-8-33(26)39(36)50(44,45)46)29-12-19-32(20-13-29)43-37-23-16-27-7-3-5-9-34(27)40(37)51(47,48)49;/h2-24,42H,41H2,1H3,(H,44,45,46)(H,47,48,49);/q;+1/p-1 | |
Source | PubChem | |
URL | https://pubchem.ncbi.nlm.nih.gov | |
Description | Data deposited in or computed by PubChem | |
InChI Key |
PCHTYMJTDHYWBP-UHFFFAOYSA-M | |
Source | PubChem | |
URL | https://pubchem.ncbi.nlm.nih.gov | |
Description | Data deposited in or computed by PubChem | |
Canonical SMILES |
CC1=C(C=CC(=C1)C(=C2C=CC(=NC3=C(C4=CC=CC=C4C=C3)S(=O)(=O)[O-])C=C2)C5=CC=C(C=C5)NC6=C(C7=CC=CC=C7C=C6)S(=O)(=O)O)N.[Na+] | |
Source | PubChem | |
URL | https://pubchem.ncbi.nlm.nih.gov | |
Description | Data deposited in or computed by PubChem | |
Molecular Formula |
C40H30N3NaO6S2 | |
Source | PubChem | |
URL | https://pubchem.ncbi.nlm.nih.gov | |
Description | Data deposited in or computed by PubChem | |
Molecular Weight |
735.8 g/mol | |
Source | PubChem | |
URL | https://pubchem.ncbi.nlm.nih.gov | |
Description | Data deposited in or computed by PubChem | |
CAS No. |
1324-86-3 | |
Record name | C.I. 42700 | |
Source | ChemIDplus | |
URL | https://pubchem.ncbi.nlm.nih.gov/substance/?source=chemidplus&sourceid=0001324863 | |
Description | ChemIDplus is a free, web search system that provides access to the structure and nomenclature authority files used for the identification of chemical substances cited in National Library of Medicine (NLM) databases, including the TOXNET system. | |
Record name | Naphthalenesulfonic acid, 2-[[4-[(4-amino-3-methylphenyl)[4-[(sulfo-2-naphthalenyl)amino]phenyl]methylene]-2,5-cyclohexadien-1-ylidene]amino]-, sodium salt (1:1) | |
Source | EPA Chemicals under the TSCA | |
URL | https://www.epa.gov/chemicals-under-tsca | |
Description | EPA Chemicals under the Toxic Substances Control Act (TSCA) collection contains information on chemicals and their regulations under TSCA, including non-confidential content from the TSCA Chemical Substance Inventory and Chemical Data Reporting. | |
Record name | Sodium hydrogen 2-[[4-[(4-amino-m-tolyl)[4-[(sulphonato-2-naphthyl)amino]phenyl]methylene]-2,5-cyclohexadien-1-ylidene]amino]naphthalene-1-sulphonate | |
Source | European Chemicals Agency (ECHA) | |
URL | https://echa.europa.eu/substance-information/-/substanceinfo/100.013.989 | |
Description | The European Chemicals Agency (ECHA) is an agency of the European Union which is the driving force among regulatory authorities in implementing the EU's groundbreaking chemicals legislation for the benefit of human health and the environment as well as for innovation and competitiveness. | |
Explanation | Use of the information, documents and data from the ECHA website is subject to the terms and conditions of this Legal Notice, and subject to other binding limitations provided for under applicable law, the information, documents and data made available on the ECHA website may be reproduced, distributed and/or used, totally or in part, for non-commercial purposes provided that ECHA is acknowledged as the source: "Source: European Chemicals Agency, http://echa.europa.eu/". Such acknowledgement must be included in each copy of the material. ECHA permits and encourages organisations and individuals to create links to the ECHA website under the following cumulative conditions: Links can only be made to webpages that provide a link to the Legal Notice page. | |
Disclaimer and Information on In-Vitro Research Products
Please be aware that all articles and product information presented on BenchChem are intended solely for informational purposes. The products available for purchase on BenchChem are specifically designed for in-vitro studies, which are conducted outside of living organisms. In-vitro studies, derived from the Latin term "in glass," involve experiments performed in controlled laboratory settings using cells or tissues. It is important to note that these products are not categorized as medicines or drugs, and they have not received approval from the FDA for the prevention, treatment, or cure of any medical condition, ailment, or disease. We must emphasize that any form of bodily introduction of these products into humans or animals is strictly prohibited by law. It is essential to adhere to these guidelines to ensure compliance with legal and ethical standards in research and experimentation.