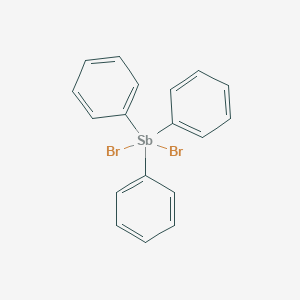
Triphenylantimony dibromide
Overview
Description
Triphenylantimony dibromide (C₁₈H₁₅Br₂Sb, molecular weight 512.89 g/mol) is a pentavalent antimony organometallic compound characterized by three phenyl groups and two bromine ligands . It is a white crystalline solid with applications in coordination chemistry, catalysis, and materials science. Its synthesis typically involves oxidative addition reactions or ligand-exchange processes, such as the reaction of triphenylantimony with bromine sources or the exchange of bromine with catechol-thioethers in the presence of triethylamine .
Structurally, this compound adopts a trigonal bipyramidal geometry, with the bromine atoms occupying axial positions and phenyl groups in equatorial positions . This arrangement creates a "sigma-hole" at the antimony center, enhancing its Lewis acidity and enabling interactions with electron-rich substrates .
Preparation Methods
Synthetic Routes and Reaction Conditions: Triphenylantimony dibromide can be synthesized through the reaction of triphenylantimony with bromine. The reaction typically occurs in an inert solvent such as benzene or toluene. The general reaction is as follows: [ \text{Ph}{3}\text{Sb} + \text{Br}{2} \rightarrow \text{Ph}{3}\text{SbBr}{2} ] where ( \text{Ph} ) represents a phenyl group.
Industrial Production Methods: In an industrial setting, the production of this compound involves the controlled addition of bromine to a solution of triphenylantimony in an inert solvent. The reaction is exothermic and requires careful temperature control to prevent decomposition of the product. The resulting this compound is then purified by recrystallization from a suitable solvent.
Chemical Reactions Analysis
Types of Reactions: Triphenylantimony dibromide undergoes various chemical reactions, including:
Substitution Reactions: It can react with nucleophiles to replace the bromine atoms with other groups. For example, it reacts with sodium propionate to form triphenylantimony dipropionate.
Oxidation-Reduction Reactions: It can participate in redox reactions, particularly involving the antimony center.
Common Reagents and Conditions:
Substitution Reactions: Common reagents include sodium salts of carboxylic acids, which react with this compound in solvents like toluene at elevated temperatures.
Oxidation-Reduction Reactions: These reactions often involve oxidizing agents such as hydrogen peroxide or reducing agents like sodium borohydride.
Major Products Formed:
Substitution Reactions: Products include various organoantimony compounds such as triphenylantimony dipropionate.
Oxidation-Reduction Reactions: Products depend on the specific redox conditions but can include antimony(V) or antimony(III) species.
Scientific Research Applications
Chemical Applications
1. Synthesis of Organometallic Compounds
Triphenylantimony dibromide is primarily used as a reagent in the synthesis of other organoantimony compounds. It serves as a precursor for creating antimony-containing polymers and coordination complexes. For instance, it can react with sodium salts of carboxylic acids to form triphenylantimony dipropionate, showcasing its versatility in organic synthesis .
2. Catalysis
In industrial applications, this compound acts as a catalyst in polymerization reactions. Its ability to facilitate chemical transformations makes it valuable in producing flame retardants and other polymeric materials .
Biological and Medicinal Applications
1. Antitumor Activity
Research indicates that organometallic compounds like this compound exhibit potential antitumor properties. Studies have shown that these compounds can influence various biochemical processes, leading to cytotoxic effects against cancer cells .
2. Antimicrobial Properties
this compound has also been explored for its antimicrobial activities. Its reactivity allows it to interact with biological targets, potentially leading to the development of new antimicrobial agents .
Case Study 1: Synthesis of Binuclear Triphenylantimony(V) Catecholates
A study focused on synthesizing binuclear triphenylantimony(V) catecholates demonstrated the compound's utility in forming complex structures through reactions with nitrogen donor ligands. The synthesis involved treating this compound with catechols under controlled conditions, resulting in stable complexes that were characterized using X-ray crystallography .
Case Study 2: Electrochemical Properties
Another investigation into the electrochemical properties of this compound revealed its role in redox reactions. The compound was shown to facilitate electron transfer processes, expanding its potential applications in electrochemical sensors and organic electronics .
Summary
This compound is a versatile compound with significant applications across chemistry, biology, and industry. Its role as a reagent in synthesizing organometallic compounds, potential antitumor and antimicrobial properties, and catalytic functions highlight its importance in scientific research and industrial processes. Ongoing studies continue to uncover new insights into its mechanisms of action and potential applications.
Mechanism of Action
The mechanism by which triphenylantimony dibromide exerts its effects involves the interaction of the antimony center with various molecular targets. In biological systems, it can interact with thiol groups in proteins, leading to the inhibition of enzyme activity. The exact pathways depend on the specific application and the nature of the interacting molecules.
Comparison with Similar Compounds
Comparison with Similar Compounds
Trimethylantimony Dibromide (C₃H₉Br₂Sb)
- Molecular Weight : 326.67 g/mol (vs. 512.89 g/mol for triphenylantimony dibromide) .
- Structure : Smaller methyl substituents reduce steric hindrance compared to bulky phenyl groups, leading to higher reactivity in ligand-exchange reactions.
- Applications : Less commonly used in catalysis due to lower thermal stability but serves as a precursor for smaller antimony complexes .
- Electrochemical Properties : Methyl groups donate less electron density to the antimony center, resulting in weaker Lewis acidity compared to this compound .
Triphenylantimony Dichloride (C₁₈H₁₅Cl₂Sb)
- Molecular Weight : 423.98 g/mol .
- Reactivity : Chloride ligands are weaker leaving groups than bromides, making this compound less effective in redox catalysis. For example, this compound outperforms the dichloride in oxidizing α-hydroxyketones due to bromine's superior redox activity .
- Structural Flexibility : Like the dibromide, it forms trigonal bipyramidal complexes but exhibits weaker intermolecular interactions (e.g., π-stacking) due to chloride’s lower polarizability .
Bis(4-Nitrophenoxy)Triphenylantimony (C₃₀H₂₄N₂O₆Sb)
- Synthesis: Derived from this compound via substitution of bromine with 4-nitrophenoxy groups .
- Applications: Used in synthesizing nitroaromatic antimony complexes for studying non-covalent interactions in crystal lattices .
- Redox Behavior : The electron-withdrawing nitro groups stabilize the antimony center, reducing its Lewis acidity compared to the dibromide .
Triphenylantimony(V) Catecholates
- Synthesis : Produced by reacting this compound with catechol-thioethers, yielding complexes with redox-active catecholate ligands .
- Redox Properties : These complexes exhibit reversible one-electron oxidation processes, a feature absent in the parent dibromide, making them useful in electrochemical applications .
- Stability : The catecholate ligand enhances thermal stability compared to the dibromide, which decomposes at lower temperatures .
Comparative Data Table
Key Research Findings
- Catalytic Efficiency: this compound enables catalytic cycles in α-hydroxyketone oxidations, achieving turnover numbers (TON) of 10–15, outperforming dichloride analogs .
- Structural Insights : X-ray crystallography reveals that bromine ligands in this compound induce stronger Sb···Br interactions (bond lengths ~2.6 Å) compared to Sb···Cl (~2.8 Å) in dichlorides, enhancing its electrophilicity .
- Thermal Stability : this compound decomposes at 180–200°C, whereas catecholate derivatives remain stable up to 250°C .
Biological Activity
Triphenylantimony dibromide (Ph₃SbBr₂) is an organometallic compound that has garnered interest for its potential biological activities, particularly in antimicrobial and anticancer applications. This article synthesizes findings from diverse studies to provide a comprehensive overview of the biological activity of this compound.
Chemical Structure and Properties
This compound consists of a central antimony atom bonded to three phenyl groups and two bromine atoms. Its unique structure allows for interactions with various biological targets, making it a subject of extensive research in medicinal chemistry.
Antimicrobial Activity
1. Antibacterial Studies
Research has demonstrated that this compound exhibits significant antibacterial properties against a range of Gram-positive and Gram-negative bacteria. A study evaluated its effectiveness against Escherichia coli, Staphylococcus aureus, and Pseudomonas aeruginosa. The results indicated that the compound showed higher inhibition zones compared to control groups, suggesting its potential as an antibacterial agent. The presence of bromine in the structure enhances its lipophilicity, which may contribute to its increased biological activity .
2. Antifungal Activity
This compound also displays antifungal properties. In vitro studies have shown activity against fungi such as Aspergillus solani and Penicillium chrysogenum. The mechanism of action appears to involve disruption of fungal cell membranes, leading to increased permeability and cell death. Comparative studies with other organoantimony compounds suggest that this compound is among the more effective derivatives .
Cytotoxicity and Antitumor Activity
The cytotoxic effects of this compound have been investigated in various cancer cell lines. Studies indicate that it can induce apoptosis in cancer cells, potentially through the generation of reactive oxygen species (ROS) and subsequent oxidative stress. For instance, experiments on breast cancer cell lines demonstrated that treatment with this compound resulted in significant reductions in cell viability, highlighting its potential as an anticancer agent .
The biological activity of this compound can be attributed to several mechanisms:
- Chelation Theory : The chelation theory suggests that metal complexes can enhance biological activity by forming stable structures with biomolecules, which can disrupt cellular processes .
- Oxidative Stress Induction : The generation of ROS can lead to oxidative damage in cells, triggering apoptosis pathways .
- Membrane Disruption : The lipophilic nature of this compound allows it to integrate into lipid membranes, causing structural changes that lead to cell lysis .
Table 1: Summary of Biological Activities
Q & A
Basic Research Questions
Q. What are the optimal synthetic routes for preparing triphenylantimony dibromide, and how do solvent systems influence yield?
this compound is synthesized via ligand substitution reactions using triphenylantimony(V) precursors. For example, reacting triphenylantimony chloride (SbPh₃Cl₂) with hydrogen bromide (HBr) in tetrahydrofuran (THF) under inert conditions yields the dibromide derivative . Solvent polarity and coordinating ability significantly affect reaction efficiency. THF, a polar aprotic solvent, enhances ion dissociation, while non-coordinating solvents like dichloromethane may reduce side reactions. Reaction monitoring via thin-layer chromatography (TLC) and purification through recrystallization from ethanol are recommended .
Q. Which spectroscopic and crystallographic methods are critical for characterizing this compound?
- X-ray crystallography resolves its trigonal bipyramidal geometry, with axial bromine ligands and equatorial phenyl groups .
- Infrared (IR) spectroscopy identifies Sb-Br stretching vibrations (~250–300 cm⁻¹) and aromatic C-H stretches (~3050 cm⁻¹).
- NMR spectroscopy (¹H, ¹³C) confirms phenyl group integrity, with aromatic proton signals at δ 7.2–7.8 ppm .
- Elemental analysis validates stoichiometry (e.g., C: 42.2%, H: 2.95%, Br: 31.1%) .
Q. How does this compound participate in ligand substitution reactions?
The bromide ligands are labile and can be replaced by stronger donors like catecholates or thiocyanate. For instance, reacting SbPh₃Br₂ with sodium thiocyanate (NaSCN) in acetone yields SbPh₃(NCS)₂, with reaction progress tracked via IR spectroscopy (disappearance of Sb-Br peaks). Stoichiometric control (1:2 molar ratio) and inert atmospheres prevent oxidation .
Advanced Research Questions
Q. What redox properties make this compound suitable for catalytic applications?
Triphenylantimony(V) derivatives exhibit reversible redox behavior, as shown by cyclic voltammetry (CV) in acetonitrile. The Sb(V)/Sb(III) couple occurs at E₁/₂ ≈ −0.45 V (vs. Ag/AgCl), enabling catalytic cycles in oxidation reactions. Binuclear complexes with bridging ligands (e.g., pyrazine) enhance redox activity by delocalizing electron density .
Q. How can thermodynamic functions of this compound inform its stability in different phases?
Low-temperature heat capacity measurements (0–480 K) reveal phase transitions and entropy changes. Debye’s multifractal model analysis (for T < 50 K) estimates the characteristic temperature (θ_D ≈ 120 K) and fractal dimension (d ≈ 2.3), suggesting a layered crystal structure. These data predict thermal stability limits (decomposition > 360°C) .
Q. What methodological challenges arise in separating this compound from reaction byproducts?
Co-distillation with unreacted bromine is a common issue. Reductive methods, such as treating the crude mixture with zinc powder, selectively reduce excess Br₂ to HBr without altering the target compound. Subsequent filtration and solvent evaporation yield pure product .
Q. How do structural modifications (e.g., substituents on phenyl rings) alter reactivity in this compound derivatives?
Electron-withdrawing groups (e.g., -NO₂) on phenyl rings increase Lewis acidity at the Sb center, accelerating ligand substitution. For example, para-nitro-substituted derivatives react with catechol 30% faster than unsubstituted analogs. Kinetic studies via UV-Vis spectroscopy and DFT calculations validate these trends .
Q. Can this compound serve as a precursor for environmentally relevant applications, such as wastewater treatment?
Its photocatalytic activity under UV light enables degradation of organic pollutants (e.g., dyes) via radical mechanisms. Experimental protocols involve dispersing SbPh₃Br₂ on TiO₂ substrates and monitoring pollutant concentration via HPLC. Controlled pH (6–8) and oxygen availability are critical for sustained activity .
Q. Data Contradictions and Resolution Strategies
- Contradiction : Conflicting reports on Sb-Br bond lengths in crystallographic studies (2.45–2.60 Å).
Resolution : Compare data collection temperatures (low-temperature studies reduce thermal motion artifacts) and refine models using high-resolution datasets . - Contradiction : Discrepancies in redox potentials (±0.1 V) across studies.
Resolution : Standardize electrolyte composition (e.g., 0.1 M TBAPF₆ in CH₃CN) and reference electrode calibration .
Properties
IUPAC Name |
dibromo(triphenyl)-λ5-stibane | |
---|---|---|
Source | PubChem | |
URL | https://pubchem.ncbi.nlm.nih.gov | |
Description | Data deposited in or computed by PubChem | |
InChI |
InChI=1S/3C6H5.2BrH.Sb/c3*1-2-4-6-5-3-1;;;/h3*1-5H;2*1H;/q;;;;;+2/p-2 | |
Source | PubChem | |
URL | https://pubchem.ncbi.nlm.nih.gov | |
Description | Data deposited in or computed by PubChem | |
InChI Key |
QHXPZUYAVMUVJN-UHFFFAOYSA-L | |
Source | PubChem | |
URL | https://pubchem.ncbi.nlm.nih.gov | |
Description | Data deposited in or computed by PubChem | |
Canonical SMILES |
C1=CC=C(C=C1)[Sb](C2=CC=CC=C2)(C3=CC=CC=C3)(Br)Br | |
Source | PubChem | |
URL | https://pubchem.ncbi.nlm.nih.gov | |
Description | Data deposited in or computed by PubChem | |
Molecular Formula |
C18H15Br2Sb | |
Source | PubChem | |
URL | https://pubchem.ncbi.nlm.nih.gov | |
Description | Data deposited in or computed by PubChem | |
DSSTOX Substance ID |
DTXSID00305981 | |
Record name | Triphenylantimonydibromide | |
Source | EPA DSSTox | |
URL | https://comptox.epa.gov/dashboard/DTXSID00305981 | |
Description | DSSTox provides a high quality public chemistry resource for supporting improved predictive toxicology. | |
Molecular Weight |
512.9 g/mol | |
Source | PubChem | |
URL | https://pubchem.ncbi.nlm.nih.gov | |
Description | Data deposited in or computed by PubChem | |
CAS No. |
1538-59-6 | |
Record name | NSC173041 | |
Source | DTP/NCI | |
URL | https://dtp.cancer.gov/dtpstandard/servlet/dwindex?searchtype=NSC&outputformat=html&searchlist=173041 | |
Description | The NCI Development Therapeutics Program (DTP) provides services and resources to the academic and private-sector research communities worldwide to facilitate the discovery and development of new cancer therapeutic agents. | |
Explanation | Unless otherwise indicated, all text within NCI products is free of copyright and may be reused without our permission. Credit the National Cancer Institute as the source. | |
Record name | Triphenylantimonydibromide | |
Source | EPA DSSTox | |
URL | https://comptox.epa.gov/dashboard/DTXSID00305981 | |
Description | DSSTox provides a high quality public chemistry resource for supporting improved predictive toxicology. | |
Record name | Triphenylantimony dibromide | |
Source | European Chemicals Agency (ECHA) | |
URL | https://echa.europa.eu/information-on-chemicals | |
Description | The European Chemicals Agency (ECHA) is an agency of the European Union which is the driving force among regulatory authorities in implementing the EU's groundbreaking chemicals legislation for the benefit of human health and the environment as well as for innovation and competitiveness. | |
Explanation | Use of the information, documents and data from the ECHA website is subject to the terms and conditions of this Legal Notice, and subject to other binding limitations provided for under applicable law, the information, documents and data made available on the ECHA website may be reproduced, distributed and/or used, totally or in part, for non-commercial purposes provided that ECHA is acknowledged as the source: "Source: European Chemicals Agency, http://echa.europa.eu/". Such acknowledgement must be included in each copy of the material. ECHA permits and encourages organisations and individuals to create links to the ECHA website under the following cumulative conditions: Links can only be made to webpages that provide a link to the Legal Notice page. | |
Retrosynthesis Analysis
AI-Powered Synthesis Planning: Our tool employs the Template_relevance Pistachio, Template_relevance Bkms_metabolic, Template_relevance Pistachio_ringbreaker, Template_relevance Reaxys, Template_relevance Reaxys_biocatalysis model, leveraging a vast database of chemical reactions to predict feasible synthetic routes.
One-Step Synthesis Focus: Specifically designed for one-step synthesis, it provides concise and direct routes for your target compounds, streamlining the synthesis process.
Accurate Predictions: Utilizing the extensive PISTACHIO, BKMS_METABOLIC, PISTACHIO_RINGBREAKER, REAXYS, REAXYS_BIOCATALYSIS database, our tool offers high-accuracy predictions, reflecting the latest in chemical research and data.
Strategy Settings
Precursor scoring | Relevance Heuristic |
---|---|
Min. plausibility | 0.01 |
Model | Template_relevance |
Template Set | Pistachio/Bkms_metabolic/Pistachio_ringbreaker/Reaxys/Reaxys_biocatalysis |
Top-N result to add to graph | 6 |
Feasible Synthetic Routes
Disclaimer and Information on In-Vitro Research Products
Please be aware that all articles and product information presented on BenchChem are intended solely for informational purposes. The products available for purchase on BenchChem are specifically designed for in-vitro studies, which are conducted outside of living organisms. In-vitro studies, derived from the Latin term "in glass," involve experiments performed in controlled laboratory settings using cells or tissues. It is important to note that these products are not categorized as medicines or drugs, and they have not received approval from the FDA for the prevention, treatment, or cure of any medical condition, ailment, or disease. We must emphasize that any form of bodily introduction of these products into humans or animals is strictly prohibited by law. It is essential to adhere to these guidelines to ensure compliance with legal and ethical standards in research and experimentation.