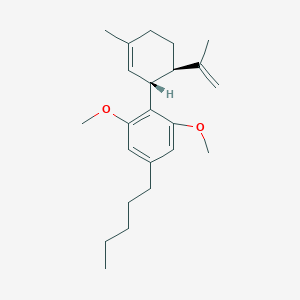
Cannabidiol dimethyl ether
Overview
Description
Cannabidiol dimethyl ether (CBDD), chemically designated as cannabidiol-2',6'-dimethyl ether, is a synthetic derivative of cannabidiol (CBD), a major non-psychoactive phytocannabinoid from Cannabis sativa. CBDD is formed by methylating the two hydroxyl groups on CBD’s resorcinol ring, resulting in a dimethyl ether structure (Fig. 1) . This modification enhances its metabolic stability and alters its pharmacological profile compared to CBD. CBDD has garnered attention for its potent and selective inhibition of 15-lipoxygenase (15-LOX), an enzyme implicated in atherosclerosis and inflammation, with an IC50 of 0.28 μM . Its unique structure-activity relationship (SAR) distinguishes it from other CBD analogs, making it a promising candidate for therapeutic development.
Preparation Methods
Synthetic Routes and Reaction Conditions: Cannabidiol dimethyl ether can be synthesized through various methods. One common approach involves the formylation of olivetol dimethyl ether, followed by a Horner-Wadsworth-Emmons reaction, DIBAL reduction, and carbonate formation . Another method involves converting olive alcohol into olive alcohol diester, followed by a coupling reaction to obtain cannabidiol diester. The diester is then purified and hydrolyzed to remove the ester groups, yielding cannabidiol .
Industrial Production Methods: Industrial production of this compound typically involves solvent extraction methods. Organic solvents such as ethanol, butane, propane, hexane, and petroleum ether are commonly used for the extraction of cannabinoids from cannabis plants . These methods ensure high yield and feasibility for large-scale production.
Chemical Reactions Analysis
Acid-Catalyzed Cyclization Reactions
CBDD undergoes intramolecular cyclization under acidic conditions, analogous to CBD, but with altered regioselectivity due to methoxy groups:
Key Findings:
-
BF₃·OEt₂ in CH₂Cl₂ at −10°C promotes cyclization to Δ⁹-THC analogs, whereas higher temperatures favor Δ⁸-THC isomers .
-
CSA (camphorsulfonic acid) in toluene at 40°C selectively yields Δ⁸-THC derivatives in high purity .
-
Methoxy groups stabilize intermediates, reducing side reactions compared to CBD .
Table 1: Cyclization Outcomes Under Selected Conditions
Acid Catalyst | Solvent | Temperature | Main Product | Yield |
---|---|---|---|---|
BF₃·OEt₂ | CH₂Cl₂ | −10°C | Δ⁹-THC dimethyl ether | 68% |
CSA | Toluene | 40°C | Δ⁸-THC dimethyl ether | 82% |
pTSA | CH₂Cl₂ | RT | Δ⁸/Δ⁹-THC mixture | 54% |
Inhibition of 15-Lipoxygenase (15-LOX)
CBDD acts as a potent inhibitor of 15-LOX-catalyzed oxidation of cholesteryl linoleate (CL), a key step in LDL oxidation:
-
IC₅₀ : 0.28 μM for 15-LOX inhibition, with 700-fold selectivity over 5-LOX .
-
Mechanism: Hydrophobic interactions within the 15-LOX binding pocket enhance inhibitory efficacy compared to CBD .
Table 2: Comparative Inhibition of 15-LOX by CBDD and CBD
Compound | IC₅₀ (μM) | Selectivity (15-LOX/5-LOX) |
---|---|---|
CBDD | 0.28 | >700 |
CBD | 12.38 | ~10 |
Dehydrogenation and Isomerization
-
Dehydrogenation of CBDD yields aromatic derivatives, as confirmed by UV spectroscopy and comparison with model compounds .
-
Isomerization under thermal or acidic conditions produces Δ⁸/Δ⁹-THC dimethyl ethers, with equilibrium favoring Δ⁸ isomers at higher temperatures .
Stability and Byproduct Formation
Scientific Research Applications
Chemical Properties and Mechanism of Action
CBDD is characterized by its unique chemical structure, which includes two dimethyl ether groups attached to the CBD backbone. This modification enhances its pharmacological properties, particularly its potency as an inhibitor of specific enzymes such as lipoxygenase (LOX), which plays a critical role in inflammatory processes .
The mechanism of action for CBDD involves interaction with various molecular targets, including cannabinoid receptors and other non-cannabinoid receptors. Its anti-inflammatory and neuroprotective effects are attributed to its ability to modulate oxidative stress and cytokine release in the central nervous system .
Neurodegenerative Disorders
CBDD has shown promise in treating neurodegenerative conditions such as Alzheimer's disease and Parkinson's disease. Preclinical studies indicate that CBDD exhibits neuroprotective effects, potentially reducing neuroinflammation and oxidative damage .
Table 1: Summary of Neuroprotective Effects of CBDD
Disorder | Mechanism of Action | Evidence Level |
---|---|---|
Alzheimer's Disease | Reduces amyloid-beta toxicity | Preclinical |
Parkinson's Disease | Modulates neuroinflammation | Preclinical |
Multiple Sclerosis | Inhibits cytokine release | Preclinical |
Anti-inflammatory Properties
As a potent LOX inhibitor, CBDD can effectively reduce inflammation, making it a candidate for treating inflammatory diseases such as arthritis and inflammatory bowel disease .
Case Study: CBDD in Arthritis Treatment
A study demonstrated that administration of CBDD significantly reduced joint inflammation and pain in animal models of arthritis, highlighting its potential as a therapeutic agent in chronic inflammatory conditions .
Antimicrobial Activity
Research indicates that extracts containing CBDD exhibit antimicrobial properties against various pathogens. A comparative study revealed that cannabis extracts using dimethyl ether as a solvent showed higher antimicrobial activity than those extracted with ethanol or butane .
Table 2: Antimicrobial Efficacy of CBDD Extracts
Pathogen | Minimum Inhibitory Concentration (MIC) | Extract Type |
---|---|---|
Staphylococcus aureus | 32 µg/mL | DME Extract |
E. coli | 64 µg/mL | DME Extract |
Candida albicans | 128 µg/mL | DME Extract |
Clinical Trials and Regulatory Status
While research on CBDD is still emerging, it is essential to note that its parent compound, CBD, has gained significant regulatory approval for specific applications, such as the treatment of epilepsy through Epidiolex® . The ongoing clinical trials investigating the efficacy of CBD derivatives like CBDD may lead to similar approvals if results demonstrate safety and effectiveness.
Mechanism of Action
Cannabidiol dimethyl ether exerts its effects primarily by inhibiting the enzyme 15-lipoxygenase. This inhibition prevents the oxygenation of linoleic acid, thereby reducing the formation of pro-inflammatory and pro-atherogenic compounds . Additionally, it may interact with various receptors and pathways, including the transient receptor potential vanilloid 1 (TRPV1) channels, G protein-coupled receptor 55 (GPR55), and peroxisome proliferator-activated receptor gamma (PPARγ) .
Comparison with Similar Compounds
Comparison with Structurally Similar Compounds
CBDD belongs to a broader class of CBD derivatives, including monomethylated analogs, homologs, and acidic precursors. Below is a comparative analysis of CBDD with key analogs:
Table 1: Structural and Functional Comparison of CBDD and Related Compounds
Key Differences:
Methylation vs. Acidic Groups : Unlike CBDA and CBDVA (cannabidivarinic acid), which possess carboxylic acid groups, CBDD’s dimethyl ether structure eliminates hydrogen-bonding capacity, enhancing lipophilicity and enzyme-binding affinity .
Side-Chain Modifications : CBDH, an n-hexyl homolog of CBD, exhibits distinct biological effects (e.g., analgesia) due to its elongated side chain, whereas CBDD’s activity arises from methylated aromatic groups .
Enzyme Selectivity : CBDD’s 15-LOX inhibition is 9-fold more potent than CBD and 4-fold more potent than CBDM, highlighting the critical role of dimethylation in enhancing potency and selectivity .
Research Findings and Mechanistic Insights
15-Lipoxygenase Inhibition
CBDD’s inhibition of 15-LOX (IC50 = 0.28 μM) is highly selective, with a 5-LOX/15-LOX IC50 ratio >700, surpassing nordihydroguaiaretic acid (NDGA), a non-selective LOX inhibitor . This selectivity is attributed to the dimethyl ether groups, which optimize steric and electronic interactions with the enzyme’s active site. In contrast, CBD’s hydroxyl groups reduce its potency (IC50 = 2.56 μM) due to weaker binding .
Receptor Modulation
CBDD shows inverse agonism at orphan receptors GPR3, GPR6, and GPR12, though with lower potency (micromolar range) compared to CBD. The free hydroxyl groups in CBD are critical for receptor interaction, as dimethylation in CBDD diminishes this activity .
Metabolic Stability
Methylation in CBDD reduces oxidative metabolism, prolonging its half-life compared to CBD. This property is advantageous for therapeutic applications requiring sustained enzyme inhibition .
Challenges and Distinctions in Identification
Structural similarities between CBDD and isomers like CBDH or CBDM complicate analytical differentiation. For instance, CBDH (n-hexyl side chain) and CBDM (monomethyl ether) exhibit nearly identical molecular weights, necessitating advanced techniques like HPLC-MS/MS with authentic standards for unambiguous identification .
Biological Activity
Cannabidiol dimethyl ether (CBDD) is a synthetic derivative of cannabidiol (CBD), which has garnered attention for its potential therapeutic properties. This article explores the biological activity of CBDD, focusing on its mechanisms of action, efficacy in various applications, and relevant research findings.
CBDD is synthesized through the methylation of the resorcinolic hydroxyl groups of CBD. This modification enhances its selectivity and potency as an inhibitor of certain biological pathways, particularly those involving lipoxygenases (LOXs), which are enzymes that catalyze the oxidation of polyunsaturated fatty acids.
Inhibition of 15-Lipoxygenase (15-LOX)
One of the most significant biological activities of CBDD is its role as a selective inhibitor of 15-LOX. In a study by Takeda et al. (2011), CBDD demonstrated an IC50 value of 0.28 μM against 15-LOX-catalyzed linoleic acid oxygenation, indicating its potent inhibitory effects compared to other cannabinoids like CBD, which had a much higher IC50 value of 12.38 μM . This inhibition is crucial in preventing the formation of oxidized low-density lipoprotein (ox-LDL), a key factor in the development of atherosclerosis.
Compound | IC50 (μM) | Selectivity Ratio (5-LOX/15-LOX) |
---|---|---|
This compound (CBDD) | 0.28 | >700 |
Cannabidiol (CBD) | 12.38 | N/A |
Antimicrobial Activity
Recent studies have highlighted the antimicrobial properties of cannabis extracts, including those obtained using dimethyl ether as a solvent. A study published in 2022 assessed the antimicrobial and antifungal activities of cannabis extracts against various pathogens, demonstrating that extracts from high-potency cannabis strains exhibited significant inhibitory effects on bacteria and dermatophytes . The minimal inhibitory concentrations (MICs) for these extracts ranged from 4 to 256 μg/mL, with dimethyl ether extracts showing superior efficacy compared to ethanol extracts.
Case Studies and Clinical Applications
Case Study: Antimicrobial Efficacy
In vitro studies have shown that CBDD and cannabis extracts can inhibit the growth of common skin pathogens, suggesting potential applications in treating dermatophytosis and other skin infections. The study indicated that DME-extracted cannabis had a higher yield of biologically active compounds compared to ethanol extracts, reinforcing the importance of extraction methods in maximizing therapeutic potential .
Case Study: Cardiovascular Health
The inhibition of ox-LDL formation by CBDD positions it as a candidate for therapeutic strategies aimed at cardiovascular diseases. By selectively targeting 15-LOX, CBDD could help mitigate oxidative stress associated with atherosclerosis, thereby supporting cardiovascular health .
Research Findings
- Selectivity and Potency : CBDD exhibits significantly higher selectivity for inhibiting 15-LOX compared to other cannabinoids, making it a valuable tool for research into lipid metabolism and oxidative stress.
- Antimicrobial Potential : The efficacy of CBDD in inhibiting microbial growth suggests its potential as an alternative or adjunctive treatment for bacterial and fungal infections.
- Therapeutic Implications : The unique properties of CBDD may lead to novel applications in treating conditions associated with oxidative stress and inflammation.
Q & A
Basic Research Questions
Q. What are the established methods for synthesizing cannabidiol dimethyl ether, and how do they differ in yield and purity?
- Methodological Answer : this compound can be synthesized via condensation reactions involving intermediates like 2-lithioorcinol dimethyl ether and menthone derivatives. For instance, Adams' approach (1940s) utilized tricyclic lactam synthesis with precursors such as olivetol dimethyl ether . Alternative routes involve nitrogen analogs of THC, where intermediates like N-benzyl-2,6-dimethoxy-4-n-amylbenzaldehyde are condensed with imine anhydrides under stereoselective conditions . Key variables affecting yield include solvent polarity, catalyst selection (e.g., acid/base catalysts), and reaction temperature. Purity is typically validated via UV/Vis spectroscopy (λmax ~284 nm) and chromatographic methods (e.g., HPLC with acetonitrile/methanol mobile phases) .
Q. How is the structural characterization of this compound performed, and what analytical techniques are critical for confirming its configuration?
- Methodological Answer : Structural elucidation relies on a combination of UV spectroscopy, nuclear magnetic resonance (NMR), and mass spectrometry. For example, UV absorption spectra (221–284 nm) are compared to model compounds to verify conjugation patterns . NMR (1H and 13C) identifies key functional groups like methyl ethers (δ 3.3–3.5 ppm) and aromatic protons. High-resolution mass spectrometry (HRMS) confirms molecular weight (e.g., C22H28O2, MW 324.5) . Discrepancies in spectral data (e.g., unexpected peaks) may indicate impurities or isomerization, necessitating recrystallization or redistillation .
Q. What safety protocols are recommended for handling this compound in laboratory settings?
- Methodological Answer : Due to limited toxicological data, researchers should treat this compound as hazardous. Protocols include:
- Using explosion-proof equipment and non-sparking tools to mitigate flammability risks .
- Conducting reactions in well-ventilated fume hoods to avoid inhalation of vapors .
- Storing solutions in inert solvents (e.g., acetonitrile) at -20°C to prevent degradation .
- Validating solvent purity via pre-concentration and chromatography to eliminate interfering residues .
Advanced Research Questions
Q. How can reaction kinetics and catalytic efficiency be optimized in the synthesis of this compound?
- Methodological Answer : Kinetic studies require a combination of fixed-bed reactor experiments and computational modeling. For example, lumped reaction kinetic models (e.g., modified Langmuir-Hinshelwood mechanisms) can be parameterized using 240+ experimental data points to predict yield under varying temperatures (150–300°C) and pressures (10–50 bar) . Catalyst selection (e.g., Cu/ZnO-ZrO2/HZSM-5 bifunctional catalysts) influences selectivity by balancing acid and metal sites . Advanced optimization employs design-of-experiment (DoE) frameworks to identify interactions between variables like CO2 co-feed ratios and space velocity .
Q. What contradictions exist in the literature regarding the pharmacological activity of this compound, and how can these be resolved experimentally?
- Methodological Answer : Contradictions arise from inconsistent purity standards and residual THC contamination in CBD derivatives. To address this:
- Implement rigorous batch-specific analysis (e.g., certificates of analysis with ≥98% purity thresholds) .
- Use gas chromatography-mass spectrometry (GC-MS) to quantify THC levels below 0.1% .
- Design controlled in vitro assays (e.g., receptor-binding studies with CB1/CB2 knockout models) to isolate dimethyl ether-specific effects .
Q. What advanced spectroscopic or computational methods are emerging for studying the stability of this compound under varying environmental conditions?
- Methodological Answer : Accelerated stability testing (e.g., 40°C/75% RH for 6 months) combined with LC-MS/MS monitors degradation products like oxidized dimers . Computational tools (e.g., density functional theory (DFT)) predict bond dissociation energies to identify vulnerable sites (e.g., ether linkages). Isotopic labeling (e.g., deuterated methyl groups) tracks degradation pathways via NMR .
Q. Data and Reproducibility
Q. How can researchers ensure reproducibility in studies involving this compound, given variability in synthetic protocols?
- Methodological Answer : Reproducibility requires:
Properties
IUPAC Name |
1,3-dimethoxy-2-[(1R,6R)-3-methyl-6-prop-1-en-2-ylcyclohex-2-en-1-yl]-5-pentylbenzene | |
---|---|---|
Source | PubChem | |
URL | https://pubchem.ncbi.nlm.nih.gov | |
Description | Data deposited in or computed by PubChem | |
InChI |
InChI=1S/C23H34O2/c1-7-8-9-10-18-14-21(24-5)23(22(15-18)25-6)20-13-17(4)11-12-19(20)16(2)3/h13-15,19-20H,2,7-12H2,1,3-6H3/t19-,20+/m0/s1 | |
Source | PubChem | |
URL | https://pubchem.ncbi.nlm.nih.gov | |
Description | Data deposited in or computed by PubChem | |
InChI Key |
UYBGHBAVRNATET-VQTJNVASSA-N | |
Source | PubChem | |
URL | https://pubchem.ncbi.nlm.nih.gov | |
Description | Data deposited in or computed by PubChem | |
Canonical SMILES |
CCCCCC1=CC(=C(C(=C1)OC)C2C=C(CCC2C(=C)C)C)OC | |
Source | PubChem | |
URL | https://pubchem.ncbi.nlm.nih.gov | |
Description | Data deposited in or computed by PubChem | |
Isomeric SMILES |
CCCCCC1=CC(=C(C(=C1)OC)[C@@H]2C=C(CC[C@H]2C(=C)C)C)OC | |
Source | PubChem | |
URL | https://pubchem.ncbi.nlm.nih.gov | |
Description | Data deposited in or computed by PubChem | |
Molecular Formula |
C23H34O2 | |
Source | PubChem | |
URL | https://pubchem.ncbi.nlm.nih.gov | |
Description | Data deposited in or computed by PubChem | |
DSSTOX Substance ID |
DTXSID00924752 | |
Record name | Cannabidiol dimethyl ether | |
Source | EPA DSSTox | |
URL | https://comptox.epa.gov/dashboard/DTXSID00924752 | |
Description | DSSTox provides a high quality public chemistry resource for supporting improved predictive toxicology. | |
Molecular Weight |
342.5 g/mol | |
Source | PubChem | |
URL | https://pubchem.ncbi.nlm.nih.gov | |
Description | Data deposited in or computed by PubChem | |
CAS No. |
1242-67-7 | |
Record name | Cannabidiol dimethyl ether | |
Source | ChemIDplus | |
URL | https://pubchem.ncbi.nlm.nih.gov/substance/?source=chemidplus&sourceid=0001242677 | |
Description | ChemIDplus is a free, web search system that provides access to the structure and nomenclature authority files used for the identification of chemical substances cited in National Library of Medicine (NLM) databases, including the TOXNET system. | |
Record name | Cannabidiol dimethyl ether | |
Source | EPA DSSTox | |
URL | https://comptox.epa.gov/dashboard/DTXSID00924752 | |
Description | DSSTox provides a high quality public chemistry resource for supporting improved predictive toxicology. | |
Record name | CANNABIDIOL DIMETHYL ETHER | |
Source | FDA Global Substance Registration System (GSRS) | |
URL | https://gsrs.ncats.nih.gov/ginas/app/beta/substances/MK7IHZ7MIM | |
Description | The FDA Global Substance Registration System (GSRS) enables the efficient and accurate exchange of information on what substances are in regulated products. Instead of relying on names, which vary across regulatory domains, countries, and regions, the GSRS knowledge base makes it possible for substances to be defined by standardized, scientific descriptions. | |
Explanation | Unless otherwise noted, the contents of the FDA website (www.fda.gov), both text and graphics, are not copyrighted. They are in the public domain and may be republished, reprinted and otherwise used freely by anyone without the need to obtain permission from FDA. Credit to the U.S. Food and Drug Administration as the source is appreciated but not required. | |
Retrosynthesis Analysis
AI-Powered Synthesis Planning: Our tool employs the Template_relevance Pistachio, Template_relevance Bkms_metabolic, Template_relevance Pistachio_ringbreaker, Template_relevance Reaxys, Template_relevance Reaxys_biocatalysis model, leveraging a vast database of chemical reactions to predict feasible synthetic routes.
One-Step Synthesis Focus: Specifically designed for one-step synthesis, it provides concise and direct routes for your target compounds, streamlining the synthesis process.
Accurate Predictions: Utilizing the extensive PISTACHIO, BKMS_METABOLIC, PISTACHIO_RINGBREAKER, REAXYS, REAXYS_BIOCATALYSIS database, our tool offers high-accuracy predictions, reflecting the latest in chemical research and data.
Strategy Settings
Precursor scoring | Relevance Heuristic |
---|---|
Min. plausibility | 0.01 |
Model | Template_relevance |
Template Set | Pistachio/Bkms_metabolic/Pistachio_ringbreaker/Reaxys/Reaxys_biocatalysis |
Top-N result to add to graph | 6 |
Feasible Synthetic Routes
Disclaimer and Information on In-Vitro Research Products
Please be aware that all articles and product information presented on BenchChem are intended solely for informational purposes. The products available for purchase on BenchChem are specifically designed for in-vitro studies, which are conducted outside of living organisms. In-vitro studies, derived from the Latin term "in glass," involve experiments performed in controlled laboratory settings using cells or tissues. It is important to note that these products are not categorized as medicines or drugs, and they have not received approval from the FDA for the prevention, treatment, or cure of any medical condition, ailment, or disease. We must emphasize that any form of bodily introduction of these products into humans or animals is strictly prohibited by law. It is essential to adhere to these guidelines to ensure compliance with legal and ethical standards in research and experimentation.