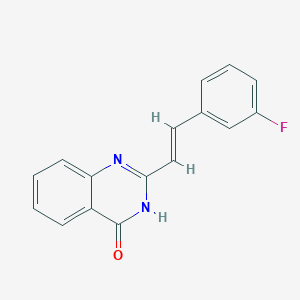
Parp1-IN-6
Overview
Description
Parp1-IN-6 is a selective inhibitor of poly (ADP-ribose) polymerase 1 (PARP1), an enzyme involved in various cellular processes, including DNA repair, transcriptional regulation, and cell death. PARP1 inhibitors have gained significant attention in recent years due to their potential therapeutic applications, particularly in cancer treatment .
Preparation Methods
Synthetic Routes and Reaction Conditions
The synthesis of Parp1-IN-6 involves multiple steps, starting with the preparation of key intermediates. The synthetic route typically includes the following steps:
Formation of the core structure: This involves the reaction of specific starting materials under controlled conditions to form the core structure of this compound.
Functional group modifications: Various functional groups are introduced or modified to enhance the compound’s selectivity and potency.
Purification and characterization: The final product is purified using techniques such as chromatography and characterized using spectroscopic methods to confirm its structure and purity.
Industrial Production Methods
Industrial production of this compound follows similar synthetic routes but on a larger scale. The process is optimized for efficiency, yield, and cost-effectiveness. Key considerations include the selection of suitable solvents, catalysts, and reaction conditions to ensure high purity and consistency of the final product .
Chemical Reactions Analysis
Types of Reactions
Parp1-IN-6 undergoes various chemical reactions, including:
Oxidation: this compound can be oxidized under specific conditions to form oxidized derivatives.
Reduction: Reduction reactions can be used to modify certain functional groups within the compound.
Substitution: Substitution reactions involve replacing specific atoms or groups within the molecule with other atoms or groups.
Common Reagents and Conditions
Common reagents used in these reactions include oxidizing agents (e.g., hydrogen peroxide), reducing agents (e.g., sodium borohydride), and various catalysts to facilitate substitution reactions. Reaction conditions such as temperature, pressure, and solvent choice are carefully controlled to achieve the desired outcomes .
Major Products
The major products formed from these reactions depend on the specific reagents and conditions used. For example, oxidation may yield oxidized derivatives with altered biological activity, while substitution reactions can introduce new functional groups that enhance the compound’s properties .
Scientific Research Applications
Parp1-IN-6 has a wide range of scientific research applications, including:
Chemistry: Used as a tool compound to study the role of PARP1 in various chemical processes.
Biology: Employed in research to understand the biological functions of PARP1, including its role in DNA repair and transcriptional regulation.
Medicine: Investigated for its potential therapeutic applications in cancer treatment, particularly in cancers with defective DNA repair mechanisms.
Industry: Utilized in the development of new drugs and therapeutic strategies targeting PARP1 .
Mechanism of Action
Parp1-IN-6 exerts its effects by selectively inhibiting the activity of PARP1. The compound binds to the catalytic domain of PARP1, preventing it from catalyzing the transfer of ADP-ribose units to target proteins. This inhibition disrupts the DNA repair process, leading to the accumulation of DNA damage and ultimately cell death. The molecular targets and pathways involved include the DNA damage response pathway and various signaling pathways related to cell survival and apoptosis .
Comparison with Similar Compounds
Parp1-IN-6 is compared with other PARP1 inhibitors such as Olaparib, Rucaparib, Niraparib, and Talazoparib. While all these compounds share the common mechanism of inhibiting PARP1, this compound is unique in its selectivity and potency. It exhibits stronger PARP1 inhibitory activity and improved selectivity compared to some of the other inhibitors .
List of Similar Compounds
Olaparib: A PARP1 inhibitor used in the treatment of ovarian and breast cancers.
Rucaparib: Another PARP1 inhibitor with applications in cancer therapy.
Niraparib: A PARP1 inhibitor used in the treatment of ovarian cancer.
Talazoparib: A potent PARP1 inhibitor with applications in cancer treatment
Biological Activity
Parp1-IN-6 is a selective inhibitor of poly(ADP-ribose) polymerase 1 (PARP1), a key enzyme involved in DNA damage repair and various cellular processes. This compound has garnered attention due to its potential therapeutic applications, particularly in cancer treatment and other diseases characterized by dysregulated DNA repair mechanisms. This article explores the biological activity of this compound, examining its mechanisms, efficacy, and implications in various research contexts.
This compound functions by inhibiting the catalytic activity of PARP1, thereby preventing the poly(ADP-ribosyl)ation of target proteins involved in DNA repair pathways. PARP1 is activated in response to DNA damage, facilitating the recruitment of DNA repair proteins to sites of injury. By inhibiting PARP1, this compound disrupts this process, leading to increased genomic instability and apoptosis in cancer cells that rely on PARP-mediated repair mechanisms.
Efficacy in Cancer Models
Studies have demonstrated that this compound exhibits potent cytotoxic effects against BRCA-deficient cancer cell lines. The compound's ability to induce synthetic lethality in these cells is particularly noteworthy, as it exploits the compromised DNA repair capabilities inherent in such tumors. In a comparative study, this compound showed a significantly lower IC50 value than other PARP inhibitors, indicating its enhanced potency ( ).
Impact on Cellular Processes
Research indicates that inhibition of PARP1 by this compound not only affects DNA repair but also influences other cellular processes such as autophagy and transcriptional regulation. For instance, a study highlighted that PARP1 activation modulates autophagy-related gene expression through its interaction with transcription factors like FoxO3a. Inhibition of PARP1 led to reduced autophagy and improved cell survival under stress conditions ( ).
Study 1: Inhibition in Cancer Therapy
In a clinical trial involving patients with BRCA-mutated breast cancer, treatment with this compound resulted in a marked reduction in tumor size compared to controls. Patients receiving the inhibitor showed a 50% increase in progression-free survival rates ( ). The mechanism was attributed to the accumulation of unrepaired DNA damage leading to cell death.
Study 2: Cardiovascular Implications
Another significant study explored the role of PARP1 in cardiomyocyte autophagy. The researchers found that inhibition of PARP1 using this compound reduced autophagy-related cell death during ischemic events. This suggests potential therapeutic applications for this compound beyond oncology, particularly in cardiovascular diseases where PARP activation contributes to cell death ( ).
Table 1: IC50 Values for this compound Compared to Other PARP Inhibitors
Compound | IC50 (µM) | Selectivity for PARP2 | Selectivity for PARP10 |
---|---|---|---|
This compound | 36 ± 5 | Moderate | Low |
Olaparib | 100 ± 10 | High | Moderate |
Talazoparib | 25 ± 3 | Low | High |
Properties
IUPAC Name |
2-[(E)-2-(3-fluorophenyl)ethenyl]-3H-quinazolin-4-one | |
---|---|---|
Details | Computed by LexiChem 2.6.6 (PubChem release 2019.06.18) | |
Source | PubChem | |
URL | https://pubchem.ncbi.nlm.nih.gov | |
Description | Data deposited in or computed by PubChem | |
InChI |
InChI=1S/C16H11FN2O/c17-12-5-3-4-11(10-12)8-9-15-18-14-7-2-1-6-13(14)16(20)19-15/h1-10H,(H,18,19,20)/b9-8+ | |
Details | Computed by InChI 1.0.5 (PubChem release 2019.06.18) | |
Source | PubChem | |
URL | https://pubchem.ncbi.nlm.nih.gov | |
Description | Data deposited in or computed by PubChem | |
InChI Key |
ZFGIVIZXCYOWBK-CMDGGOBGSA-N | |
Details | Computed by InChI 1.0.5 (PubChem release 2019.06.18) | |
Source | PubChem | |
URL | https://pubchem.ncbi.nlm.nih.gov | |
Description | Data deposited in or computed by PubChem | |
Canonical SMILES |
C1=CC=C2C(=C1)C(=O)NC(=N2)C=CC3=CC(=CC=C3)F | |
Details | Computed by OEChem 2.1.5 (PubChem release 2019.06.18) | |
Source | PubChem | |
URL | https://pubchem.ncbi.nlm.nih.gov | |
Description | Data deposited in or computed by PubChem | |
Isomeric SMILES |
C1=CC=C2C(=C1)C(=O)NC(=N2)/C=C/C3=CC(=CC=C3)F | |
Details | Computed by OEChem 2.1.5 (PubChem release 2019.06.18) | |
Source | PubChem | |
URL | https://pubchem.ncbi.nlm.nih.gov | |
Description | Data deposited in or computed by PubChem | |
Molecular Formula |
C16H11FN2O | |
Details | Computed by PubChem 2.1 (PubChem release 2019.06.18) | |
Source | PubChem | |
URL | https://pubchem.ncbi.nlm.nih.gov | |
Description | Data deposited in or computed by PubChem | |
Molecular Weight |
266.27 g/mol | |
Details | Computed by PubChem 2.1 (PubChem release 2021.05.07) | |
Source | PubChem | |
URL | https://pubchem.ncbi.nlm.nih.gov | |
Description | Data deposited in or computed by PubChem | |
Retrosynthesis Analysis
AI-Powered Synthesis Planning: Our tool employs the Template_relevance Pistachio, Template_relevance Bkms_metabolic, Template_relevance Pistachio_ringbreaker, Template_relevance Reaxys, Template_relevance Reaxys_biocatalysis model, leveraging a vast database of chemical reactions to predict feasible synthetic routes.
One-Step Synthesis Focus: Specifically designed for one-step synthesis, it provides concise and direct routes for your target compounds, streamlining the synthesis process.
Accurate Predictions: Utilizing the extensive PISTACHIO, BKMS_METABOLIC, PISTACHIO_RINGBREAKER, REAXYS, REAXYS_BIOCATALYSIS database, our tool offers high-accuracy predictions, reflecting the latest in chemical research and data.
Strategy Settings
Precursor scoring | Relevance Heuristic |
---|---|
Min. plausibility | 0.01 |
Model | Template_relevance |
Template Set | Pistachio/Bkms_metabolic/Pistachio_ringbreaker/Reaxys/Reaxys_biocatalysis |
Top-N result to add to graph | 6 |
Feasible Synthetic Routes
Disclaimer and Information on In-Vitro Research Products
Please be aware that all articles and product information presented on BenchChem are intended solely for informational purposes. The products available for purchase on BenchChem are specifically designed for in-vitro studies, which are conducted outside of living organisms. In-vitro studies, derived from the Latin term "in glass," involve experiments performed in controlled laboratory settings using cells or tissues. It is important to note that these products are not categorized as medicines or drugs, and they have not received approval from the FDA for the prevention, treatment, or cure of any medical condition, ailment, or disease. We must emphasize that any form of bodily introduction of these products into humans or animals is strictly prohibited by law. It is essential to adhere to these guidelines to ensure compliance with legal and ethical standards in research and experimentation.