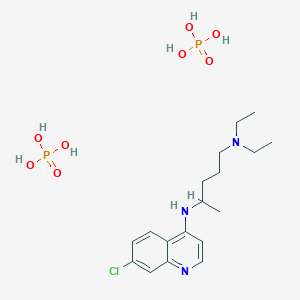
Chloroquine phosphate
Overview
Description
Mechanism of Action
Target of Action
Chloroquine phosphate, an antimalarial drug, primarily targets the asexual form of the malaria parasite during its life cycle within the red blood cell . It is particularly effective against Plasmodium vivax, P. malariae, P. ovale, and susceptible strains of P. falciparum . The drug also exhibits activity against the trophozoites of Entamoeba histolytica .
Mode of Action
Chloroquine inhibits the action of heme polymerase in malarial trophozoites, preventing the conversion of heme to hemazoin . This results in the accumulation of toxic heme, which is lethal to the parasite . Chloroquine also binds to and inhibits DNA and RNA polymerase, interferes with the metabolism and hemoglobin utilization by parasites, and inhibits prostaglandin effects . It concentrates within parasite acid vesicles and raises internal pH, resulting in inhibition of parasite growth .
Biochemical Pathways
Chloroquine affects several biochemical pathways. It has been found to inactivate the autophagy pathway, which is a potential target in chemotherapy-resistant patients . By down-regulating the autophagy pathway, chloroquine can exert a synergistic antitumor effect with chemotherapy drugs . Furthermore, it has been observed that chloroquine can aggravate lipid accumulation and reduce intracellular energy synthesis in hepatocellular carcinoma cells .
Pharmacokinetics
Chloroquine is rapidly and almost completely absorbed from the gastrointestinal tract, and only a small proportion of the administered dose is found in the stools . Approximately 55% of the drug in the plasma is bound to nondiffusible plasma constituents . Excretion of chloroquine is quite slow, but is increased by acidification of the urine . Chloroquine is deposited in the tissues in considerable amounts, with the liver, spleen, kidney, and lung containing from 200 to 700 times the plasma concentration . The main metabolite is desethylchloroquine, which accounts for one fourth of the total material appearing in the urine .
Result of Action
The molecular and cellular effects of chloroquine’s action are diverse. In the context of malaria, the accumulation of toxic heme leads to the death of the parasite . In cancer cells, chloroquine has been found to inhibit cell proliferation, as evidenced by a decrease in cellular ATP content, Ki-67 and S6K1 protein expression, and a reduction in cell number . This is associated with an increase in lipid content . Chloroquine also induces a phospholipidosis-like phenotype, characterized by the appearance of numerous multivesicular and multilamellar bodies within the lumen of expanded cytoplasmic vesicles .
Action Environment
The action, efficacy, and stability of chloroquine can be influenced by environmental factors. For instance, the anaerobic environment can avoid the competitive adsorption and consumption behavior of dissolved O2 and PMS on the photocatalyst surface, thus maximizing the transfer of surface photogenerated charges to PMS for PMS activation and active species generation . This can lead to the efficient degradation of this compound .
Biochemical Analysis
Biochemical Properties
Chloroquine phosphate interacts with various enzymes, proteins, and other biomolecules. It has exhibited a broad spectrum of action against various fungus, bacteria, and viruses . High-performance liquid chromatography (HPLC) coupled to UV detectors is the most employed method to quantify this compound in pharmaceutical products and biological samples .
Cellular Effects
This compound has wide-ranging effects on various types of cells and cellular processes. It influences cell function, including impact on cell signaling pathways, gene expression, and cellular metabolism .
Molecular Mechanism
The molecular mechanism of action of this compound involves binding interactions with biomolecules, enzyme inhibition or activation, and changes in gene expression .
Temporal Effects in Laboratory Settings
In laboratory settings, the effects of this compound change over time. Information on the product’s stability, degradation, and any long-term effects on cellular function observed in in vitro or in vivo studies is crucial .
Dosage Effects in Animal Models
The effects of this compound vary with different dosages in animal models. This includes any threshold effects observed in these studies, as well as any toxic or adverse effects at high doses .
Metabolic Pathways
This compound is involved in various metabolic pathways, interacting with enzymes or cofactors. It can also affect metabolic flux or metabolite levels .
Transport and Distribution
This compound is transported and distributed within cells and tissues. This includes any transporters or binding proteins that it interacts with, as well as any effects on its localization or accumulation .
Subcellular Localization
The subcellular localization of this compound and any effects on its activity or function are important. This includes any targeting signals or post-translational modifications that direct it to specific compartments or organelles .
Preparation Methods
Synthetic Routes and Reaction Conditions: The synthesis of chloroquine phosphate involves the reaction of 4,7-dichloroquinoline with 4-diethylamino-1-methylbutylamine. The reaction typically occurs in the presence of a base such as sodium hydroxide or potassium carbonate, and the product is then converted to its phosphate salt form by reaction with phosphoric acid .
Industrial Production Methods: Industrial production of this compound follows a similar synthetic route but on a larger scale. The process involves stringent quality control measures to ensure the purity and efficacy of the final product. The crystalline substance is then formulated into tablets or other dosage forms for medical use .
Types of Reactions:
Reduction: Although less common, chloroquine can also participate in reduction reactions under specific conditions.
Substitution: Chloroquine can undergo substitution reactions, particularly at the amino group, leading to the formation of various derivatives.
Common Reagents and Conditions:
Oxidation: Common oxidizing agents include hydrogen peroxide and potassium permanganate.
Reduction: Reducing agents such as sodium borohydride can be used.
Substitution: Substitution reactions often involve reagents like alkyl halides or acyl chlorides.
Major Products Formed:
Desethylchloroquine: A primary metabolite formed through oxidation.
Bisdesethylchloroquine: Another metabolite formed through further oxidation.
Scientific Research Applications
Aralen phosphate has a wide range of scientific research applications:
Chemistry: Used as a reagent in various chemical reactions and studies involving DNA intercalation.
Medicine: Primarily used for the treatment and prevention of malaria and amebiasis.
Industry: Utilized in the production of antimalarial drugs and other pharmaceutical formulations.
Comparison with Similar Compounds
Quinine: Another antimalarial drug with a similar mechanism of action.
Hydroxychloroquine: A derivative of chloroquine with similar uses but a different safety profile.
Mefloquine: An antimalarial drug with a different chemical structure but similar therapeutic effects.
Uniqueness of Aralen Phosphate: Aralen phosphate is unique due to its specific chemical structure, which includes a chlorine atom at the seventh position of the quinoline ring. This structural feature is crucial for its antimalarial activity . Additionally, its ability to inhibit heme polymerase and interact with DNA sets it apart from other antimalarial drugs .
Properties
CAS No. |
50-63-5 |
---|---|
Molecular Formula |
C18H29ClN3O4P |
Molecular Weight |
417.9 g/mol |
IUPAC Name |
4-N-(7-chloroquinolin-4-yl)-1-N,1-N-diethylpentane-1,4-diamine;phosphoric acid |
InChI |
InChI=1S/C18H26ClN3.H3O4P/c1-4-22(5-2)12-6-7-14(3)21-17-10-11-20-18-13-15(19)8-9-16(17)18;1-5(2,3)4/h8-11,13-14H,4-7,12H2,1-3H3,(H,20,21);(H3,1,2,3,4) |
InChI Key |
AEUAEICGCMSYCQ-UHFFFAOYSA-N |
SMILES |
CCN(CC)CCCC(C)NC1=C2C=CC(=CC2=NC=C1)Cl.OP(=O)(O)O.OP(=O)(O)O |
Canonical SMILES |
CCN(CC)CCCC(C)NC1=C2C=CC(=CC2=NC=C1)Cl.OP(=O)(O)O |
Key on ui other cas no. |
50-63-5 |
Pictograms |
Irritant; Health Hazard |
Purity |
98% |
Related CAS |
69698-56-2, 6384-82-3 |
Synonyms |
arechin; chingamin phosphate; chloroquine bis(dihydrogenphosphate) dihydrate; chloroquine diphosphate; chloroquine diphosphate, (+)-isomer; chloroquine diphosphate, (+-)-isomer; chloroquine diphosphate, (-)-isomer; chloroquine phosphate; delagil; khingamin phosphate; Resochin; unspecified phosphate of chloroquine diphosphate |
Origin of Product |
United States |
Retrosynthesis Analysis
AI-Powered Synthesis Planning: Our tool employs the Template_relevance Pistachio, Template_relevance Bkms_metabolic, Template_relevance Pistachio_ringbreaker, Template_relevance Reaxys, Template_relevance Reaxys_biocatalysis model, leveraging a vast database of chemical reactions to predict feasible synthetic routes.
One-Step Synthesis Focus: Specifically designed for one-step synthesis, it provides concise and direct routes for your target compounds, streamlining the synthesis process.
Accurate Predictions: Utilizing the extensive PISTACHIO, BKMS_METABOLIC, PISTACHIO_RINGBREAKER, REAXYS, REAXYS_BIOCATALYSIS database, our tool offers high-accuracy predictions, reflecting the latest in chemical research and data.
Strategy Settings
Precursor scoring | Relevance Heuristic |
---|---|
Min. plausibility | 0.01 |
Model | Template_relevance |
Template Set | Pistachio/Bkms_metabolic/Pistachio_ringbreaker/Reaxys/Reaxys_biocatalysis |
Top-N result to add to graph | 6 |
Feasible Synthetic Routes
Disclaimer and Information on In-Vitro Research Products
Please be aware that all articles and product information presented on BenchChem are intended solely for informational purposes. The products available for purchase on BenchChem are specifically designed for in-vitro studies, which are conducted outside of living organisms. In-vitro studies, derived from the Latin term "in glass," involve experiments performed in controlled laboratory settings using cells or tissues. It is important to note that these products are not categorized as medicines or drugs, and they have not received approval from the FDA for the prevention, treatment, or cure of any medical condition, ailment, or disease. We must emphasize that any form of bodily introduction of these products into humans or animals is strictly prohibited by law. It is essential to adhere to these guidelines to ensure compliance with legal and ethical standards in research and experimentation.