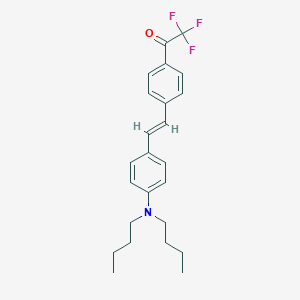
Chromoionophore IX
Overview
Description
Scientific Research Applications
Chromoionophore IX has a wide range of scientific research applications, including:
Mechanism of Action
Future Directions
Recent advances aim to renovate the chromoionophores and detection modes to overcome the pH cross-response and to eliminate the background optical interference . Topics include sensors based on solvatochromic dyes, alternative chromoionophores, photoswitchable sensors, upconverting nanoparticles, luminescence decay time, and others . Another promising direction is the development of wearable chemical sensors, where Chromoionophore IX could be used for monitoring sweat composition .
Preparation Methods
Synthetic Routes and Reaction Conditions
Chromoionophore IX can be synthesized through a series of chemical reactions involving the condensation of 4-dibutylaminobenzaldehyde with 4-trifluoroacetylbenzaldehyde . The reaction typically requires a base catalyst and is carried out under controlled temperature conditions to ensure the formation of the desired product .
Industrial Production Methods
While specific industrial production methods for this compound are not widely documented, the synthesis generally follows the same principles as laboratory preparation, with scaling up of the reaction conditions and optimization for higher yields and purity .
Chemical Reactions Analysis
Types of Reactions
Chromoionophore IX undergoes various types of chemical reactions, including:
Oxidation: The compound can be oxidized under specific conditions, leading to changes in its fluorescence properties.
Reduction: Reduction reactions can also alter the fluorescence and absorbance characteristics of this compound.
Substitution: The compound can participate in substitution reactions, particularly with alcohols, which affect its optical properties.
Common Reagents and Conditions
Common reagents used in reactions with this compound include oxidizing agents, reducing agents, and alcohols . The reactions are typically carried out under controlled temperature and pH conditions to ensure the desired outcomes .
Major Products Formed
The major products formed from reactions involving this compound depend on the specific reaction conditions and reagents used. For example, oxidation and reduction reactions can lead to different fluorescent derivatives .
Comparison with Similar Compounds
Similar Compounds
- Chromoionophore I
- Chromoionophore II
- Chromoionophore III
- Chromoionophore IV
- Chromoionophore VI
- Chromoionophore VII
- Chromoionophore VIII
- Chromoionophore XVII
Uniqueness
Chromoionophore IX is unique due to its specific interaction with alcohols, which results in distinct changes in fluorescence and absorbance . This property makes it particularly useful in the development of optical sensors for detecting alcohols, setting it apart from other chromoionophores .
Properties
IUPAC Name |
1-[4-[(E)-2-[4-(dibutylamino)phenyl]ethenyl]phenyl]-2,2,2-trifluoroethanone | |
---|---|---|
Source | PubChem | |
URL | https://pubchem.ncbi.nlm.nih.gov | |
Description | Data deposited in or computed by PubChem | |
InChI |
InChI=1S/C24H28F3NO/c1-3-5-17-28(18-6-4-2)22-15-11-20(12-16-22)8-7-19-9-13-21(14-10-19)23(29)24(25,26)27/h7-16H,3-6,17-18H2,1-2H3/b8-7+ | |
Source | PubChem | |
URL | https://pubchem.ncbi.nlm.nih.gov | |
Description | Data deposited in or computed by PubChem | |
InChI Key |
HVWDIHBWRAWRHS-BQYQJAHWSA-N | |
Source | PubChem | |
URL | https://pubchem.ncbi.nlm.nih.gov | |
Description | Data deposited in or computed by PubChem | |
Canonical SMILES |
CCCCN(CCCC)C1=CC=C(C=C1)C=CC2=CC=C(C=C2)C(=O)C(F)(F)F | |
Source | PubChem | |
URL | https://pubchem.ncbi.nlm.nih.gov | |
Description | Data deposited in or computed by PubChem | |
Isomeric SMILES |
CCCCN(CCCC)C1=CC=C(C=C1)/C=C/C2=CC=C(C=C2)C(=O)C(F)(F)F | |
Source | PubChem | |
URL | https://pubchem.ncbi.nlm.nih.gov | |
Description | Data deposited in or computed by PubChem | |
Molecular Formula |
C24H28F3NO | |
Source | PubChem | |
URL | https://pubchem.ncbi.nlm.nih.gov | |
Description | Data deposited in or computed by PubChem | |
DSSTOX Substance ID |
DTXSID30421749 | |
Record name | Chromoionophore IX | |
Source | EPA DSSTox | |
URL | https://comptox.epa.gov/dashboard/DTXSID30421749 | |
Description | DSSTox provides a high quality public chemistry resource for supporting improved predictive toxicology. | |
Molecular Weight |
403.5 g/mol | |
Source | PubChem | |
URL | https://pubchem.ncbi.nlm.nih.gov | |
Description | Data deposited in or computed by PubChem | |
CAS No. |
192190-91-3 | |
Record name | Chromoionophore IX | |
Source | EPA DSSTox | |
URL | https://comptox.epa.gov/dashboard/DTXSID30421749 | |
Description | DSSTox provides a high quality public chemistry resource for supporting improved predictive toxicology. | |
Retrosynthesis Analysis
AI-Powered Synthesis Planning: Our tool employs the Template_relevance Pistachio, Template_relevance Bkms_metabolic, Template_relevance Pistachio_ringbreaker, Template_relevance Reaxys, Template_relevance Reaxys_biocatalysis model, leveraging a vast database of chemical reactions to predict feasible synthetic routes.
One-Step Synthesis Focus: Specifically designed for one-step synthesis, it provides concise and direct routes for your target compounds, streamlining the synthesis process.
Accurate Predictions: Utilizing the extensive PISTACHIO, BKMS_METABOLIC, PISTACHIO_RINGBREAKER, REAXYS, REAXYS_BIOCATALYSIS database, our tool offers high-accuracy predictions, reflecting the latest in chemical research and data.
Strategy Settings
Precursor scoring | Relevance Heuristic |
---|---|
Min. plausibility | 0.01 |
Model | Template_relevance |
Template Set | Pistachio/Bkms_metabolic/Pistachio_ringbreaker/Reaxys/Reaxys_biocatalysis |
Top-N result to add to graph | 6 |
Feasible Synthetic Routes
Q1: How compatible is Chromoionophore IX with ethyl cellulose as a material for thin-film sensor fabrication?
A3: The research indicates positive material compatibility between this compound and ethyl cellulose []. The study successfully fabricated thin-film sensors by dissolving this compound in ethyl cellulose and then depositing this solution onto glass slides via spin-coating []. This successful fabrication suggests that this compound demonstrates sufficient solubility and stability in ethyl cellulose to form a homogenous and functional sensing layer.
Disclaimer and Information on In-Vitro Research Products
Please be aware that all articles and product information presented on BenchChem are intended solely for informational purposes. The products available for purchase on BenchChem are specifically designed for in-vitro studies, which are conducted outside of living organisms. In-vitro studies, derived from the Latin term "in glass," involve experiments performed in controlled laboratory settings using cells or tissues. It is important to note that these products are not categorized as medicines or drugs, and they have not received approval from the FDA for the prevention, treatment, or cure of any medical condition, ailment, or disease. We must emphasize that any form of bodily introduction of these products into humans or animals is strictly prohibited by law. It is essential to adhere to these guidelines to ensure compliance with legal and ethical standards in research and experimentation.