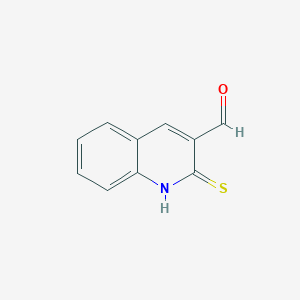
2-Mercaptoquinoline-3-carbaldehyde
Overview
Description
2-Mercaptoquinoline-3-carbaldehyde is an organic compound that belongs to the quinoline family It is characterized by the presence of a mercapto group (-SH) at the second position and an aldehyde group (-CHO) at the third position of the quinoline ring
Mechanism of Action
Target of Action
The primary target of 2-Mercaptoquinoline-3-carbaldehyde is the enzyme α-glucosidase . This enzyme catalyzes the hydrolysis of starch in the intestine, converting carbohydrates into monosaccharides to provide energy for the human body .
Mode of Action
This compound interacts with α-glucosidase through a series of reactions. The compound is involved in a three-component reaction catalyzed by L-proline, leading to the synthesis of various 4H-substituted thiopyrano[2,3-b]quinolines derivatives via a Knoevenagel condensation followed by inter-intramolecular double Michael addition reaction .
Biochemical Pathways
The biochemical pathway affected by this compound involves the inhibition of α-glucosidase. This mechanism is particularly relevant in the management of type-2 diabetes, where controlling hyperglycemia is crucial .
Pharmacokinetics
The most active derivative of this compound exhibits a competitive mode of inhibition with a Ki value of 18.0 µM .
Result of Action
The molecular and cellular effects of this compound’s action are significant. The compound’s interaction with α-glucosidase leads to a decrease in the enzyme’s activity . This results in a reduction in the rate of carbohydrate digestion, thereby lowering postprandial blood glucose levels .
Action Environment
The action of this compound can be influenced by various environmental factors. For instance, the reaction conditions, such as the presence of L-proline and the type of solvent used, can affect the compound’s synthesis and subsequent interaction with α-glucosidase
Biochemical Analysis
Biochemical Properties
2-Mercaptoquinoline-3-carbaldehyde plays a crucial role in biochemical reactions, particularly as an inhibitor of specific enzymes. One of the primary enzymes it interacts with is α-glucosidase, an enzyme involved in the hydrolysis of carbohydrates. Studies have shown that this compound exhibits potent inhibitory activity against α-glucosidase, with an IC50 value significantly lower than that of standard inhibitors . The interaction between this compound and α-glucosidase involves several hydrogen bonds, hydrophobic, and hydrophilic interactions, which interfere with the enzyme’s catalytic potential . Additionally, this compound has been found to interact with other biomolecules, including proteins and nucleic acids, further highlighting its versatility in biochemical applications.
Cellular Effects
The effects of this compound on various types of cells and cellular processes are profound. In cancer cell lines, such as melanoma, this compound has demonstrated antiproliferative effects by inhibiting the epidermal growth factor receptor (EGFR) tyrosine kinase . This inhibition leads to cell cycle arrest at the G2/M phase and induces apoptosis in pre-G1 phase . Furthermore, this compound influences cell signaling pathways, gene expression, and cellular metabolism, making it a potential candidate for cancer therapy. Its impact on cell function is also evident in its ability to act as a biosensing and bioimaging agent, with negligible toxicity towards mammalian cells .
Molecular Mechanism
The molecular mechanism of action of this compound involves its binding interactions with various biomolecules. The compound’s mercapto group allows it to form covalent bonds with thiol groups in proteins, leading to enzyme inhibition or activation . In the case of α-glucosidase, this compound binds competitively to the enzyme’s active site, thereby reducing its activity . Additionally, the aldehyde group of this compound can form Schiff bases with amino groups in proteins, further modulating their function. These interactions result in changes in gene expression and cellular metabolism, contributing to the compound’s overall biochemical effects.
Temporal Effects in Laboratory Settings
In laboratory settings, the effects of this compound have been observed to change over time. The compound exhibits good stability under standard laboratory conditions, but its activity can be influenced by factors such as pH, temperature, and the presence of other reactive species . Over extended periods, this compound may undergo degradation, leading to a decrease in its inhibitory activity . Long-term studies have shown that the compound can have sustained effects on cellular function, particularly in in vitro and in vivo models, where it continues to inhibit enzyme activity and modulate cellular processes over time .
Dosage Effects in Animal Models
The effects of this compound vary with different dosages in animal models. At lower doses, the compound has been shown to effectively inhibit target enzymes without causing significant toxicity . At higher doses, this compound can exhibit toxic effects, including hepatotoxicity and nephrotoxicity . Threshold effects have been observed, where the compound’s efficacy plateaus beyond a certain dosage, indicating the importance of optimizing dosage levels for therapeutic applications . These findings underscore the need for careful dosage management in preclinical and clinical studies involving this compound.
Metabolic Pathways
This compound is involved in several metabolic pathways, primarily through its interactions with enzymes and cofactors. The compound’s inhibitory effect on α-glucosidase impacts carbohydrate metabolism by reducing the hydrolysis of carbohydrates into monosaccharides . This inhibition leads to a decrease in postprandial blood glucose levels, making this compound a potential candidate for managing diabetes mellitus . Additionally, the compound’s interactions with other enzymes and cofactors can influence metabolic flux and alter metabolite levels, further highlighting its role in metabolic regulation .
Transport and Distribution
The transport and distribution of this compound within cells and tissues are facilitated by specific transporters and binding proteins. The compound’s mercapto group allows it to interact with thiol-containing transporters, aiding its cellular uptake and distribution . Once inside the cell, this compound can localize to various cellular compartments, including the cytoplasm and nucleus . Its distribution is influenced by factors such as concentration gradients, binding affinities, and the presence of competing molecules . These interactions determine the compound’s localization and accumulation within cells and tissues, impacting its overall biochemical activity.
Subcellular Localization
The subcellular localization of this compound plays a crucial role in its activity and function. The compound is known to localize to specific compartments within the cell, including the mitochondria and endoplasmic reticulum . Targeting signals and post-translational modifications, such as phosphorylation and acetylation, can direct this compound to these organelles, where it exerts its effects . The compound’s localization to the mitochondria, for example, can influence mitochondrial function and energy metabolism, while its presence in the endoplasmic reticulum can affect protein folding and stress responses . These subcellular interactions are critical for understanding the comprehensive biochemical effects of this compound.
Preparation Methods
Synthetic Routes and Reaction Conditions
The synthesis of 2-mercaptoquinoline-3-carbaldehyde typically involves the reaction of quinoline derivatives with sulfur-containing reagents. One common method is the condensation of 2-mercaptoaniline with formylating agents under controlled conditions. The reaction is often carried out in the presence of catalysts such as piperidine, pyridine, or triethylamine, and solvents like ethanol or methanol .
Industrial Production Methods
Industrial production of this compound may involve large-scale synthesis using similar methods as in laboratory settings but optimized for higher yields and purity. The use of continuous flow reactors and automated systems can enhance the efficiency and scalability of the production process.
Chemical Reactions Analysis
Types of Reactions
2-Mercaptoquinoline-3-carbaldehyde undergoes various types of chemical reactions, including:
Oxidation: The mercapto group can be oxidized to form disulfides or sulfonic acids.
Reduction: The aldehyde group can be reduced to form alcohols.
Substitution: The hydrogen atoms on the quinoline ring can be substituted with various functional groups.
Common Reagents and Conditions
Oxidation: Common oxidizing agents include hydrogen peroxide and potassium permanganate.
Reduction: Reducing agents such as sodium borohydride or lithium aluminum hydride are often used.
Substitution: Reagents like halogens, alkylating agents, and nitrating agents are used under specific conditions to achieve substitution reactions.
Major Products Formed
Oxidation: Disulfides, sulfonic acids.
Reduction: Alcohols.
Substitution: Halogenated, alkylated, or nitrated quinoline derivatives.
Scientific Research Applications
2-Mercaptoquinoline-3-carbaldehyde has a wide range of applications in scientific research:
Chemistry: Used as a building block in the synthesis of complex organic molecules and heterocyclic compounds.
Industry: Utilized in the production of dyes, pigments, and other specialty chemicals.
Comparison with Similar Compounds
Similar Compounds
2-Chloroquinoline-3-carbaldehyde: Similar structure but with a chloro group instead of a mercapto group.
2-Hydroxyquinoline-3-carbaldehyde: Contains a hydroxy group instead of a mercapto group.
2-Aminoquinoline-3-carbaldehyde: Features an amino group in place of the mercapto group.
Uniqueness
2-Mercaptoquinoline-3-carbaldehyde is unique due to the presence of the mercapto group, which imparts distinct chemical reactivity and biological properties. This makes it a valuable compound for specific applications where sulfur-containing functional groups are advantageous .
Properties
IUPAC Name |
2-sulfanylidene-1H-quinoline-3-carbaldehyde | |
---|---|---|
Details | Computed by LexiChem 2.6.6 (PubChem release 2019.06.18) | |
Source | PubChem | |
URL | https://pubchem.ncbi.nlm.nih.gov | |
Description | Data deposited in or computed by PubChem | |
InChI |
InChI=1S/C10H7NOS/c12-6-8-5-7-3-1-2-4-9(7)11-10(8)13/h1-6H,(H,11,13) | |
Details | Computed by InChI 1.0.5 (PubChem release 2019.06.18) | |
Source | PubChem | |
URL | https://pubchem.ncbi.nlm.nih.gov | |
Description | Data deposited in or computed by PubChem | |
InChI Key |
QGNIZTZEZZJHEK-UHFFFAOYSA-N | |
Details | Computed by InChI 1.0.5 (PubChem release 2019.06.18) | |
Source | PubChem | |
URL | https://pubchem.ncbi.nlm.nih.gov | |
Description | Data deposited in or computed by PubChem | |
Canonical SMILES |
C1=CC=C2C(=C1)C=C(C(=S)N2)C=O | |
Details | Computed by OEChem 2.1.5 (PubChem release 2019.06.18) | |
Source | PubChem | |
URL | https://pubchem.ncbi.nlm.nih.gov | |
Description | Data deposited in or computed by PubChem | |
Molecular Formula |
C10H7NOS | |
Details | Computed by PubChem 2.1 (PubChem release 2019.06.18) | |
Source | PubChem | |
URL | https://pubchem.ncbi.nlm.nih.gov | |
Description | Data deposited in or computed by PubChem | |
DSSTOX Substance ID |
DTXSID70365350 | |
Record name | 2-Sulfanylidene-1,2-dihydroquinoline-3-carbaldehyde | |
Source | EPA DSSTox | |
URL | https://comptox.epa.gov/dashboard/DTXSID70365350 | |
Description | DSSTox provides a high quality public chemistry resource for supporting improved predictive toxicology. | |
Molecular Weight |
189.24 g/mol | |
Details | Computed by PubChem 2.1 (PubChem release 2021.05.07) | |
Source | PubChem | |
URL | https://pubchem.ncbi.nlm.nih.gov | |
Description | Data deposited in or computed by PubChem | |
CAS No. |
51925-41-8 | |
Record name | 2-Sulfanylidene-1,2-dihydroquinoline-3-carbaldehyde | |
Source | EPA DSSTox | |
URL | https://comptox.epa.gov/dashboard/DTXSID70365350 | |
Description | DSSTox provides a high quality public chemistry resource for supporting improved predictive toxicology. | |
Retrosynthesis Analysis
AI-Powered Synthesis Planning: Our tool employs the Template_relevance Pistachio, Template_relevance Bkms_metabolic, Template_relevance Pistachio_ringbreaker, Template_relevance Reaxys, Template_relevance Reaxys_biocatalysis model, leveraging a vast database of chemical reactions to predict feasible synthetic routes.
One-Step Synthesis Focus: Specifically designed for one-step synthesis, it provides concise and direct routes for your target compounds, streamlining the synthesis process.
Accurate Predictions: Utilizing the extensive PISTACHIO, BKMS_METABOLIC, PISTACHIO_RINGBREAKER, REAXYS, REAXYS_BIOCATALYSIS database, our tool offers high-accuracy predictions, reflecting the latest in chemical research and data.
Strategy Settings
Precursor scoring | Relevance Heuristic |
---|---|
Min. plausibility | 0.01 |
Model | Template_relevance |
Template Set | Pistachio/Bkms_metabolic/Pistachio_ringbreaker/Reaxys/Reaxys_biocatalysis |
Top-N result to add to graph | 6 |
Feasible Synthetic Routes
Q1: What are the primary applications of 2-Mercaptoquinoline-3-carbaldehyde in synthetic organic chemistry?
A1: this compound serves as a versatile building block in synthesizing diverse heterocyclic compounds. Its reactivity stems from the presence of three reactive functional groups: the aldehyde, mercapto, and quinoline moieties. Researchers have utilized this compound in various reactions, including:
- Synthesis of thiopyrano[2,3-b]quinoline derivatives: This is achieved through multi-component reactions involving this compound, dialkyl acetylenedicarboxylates, and triphenylphosphine [, ]. This reaction efficiently generates a tricyclic system with potential biological applications.
- Construction of thiochromenoquinoline-fused polyheterocycles: This involves a domino Knoevenagel/hetero-Diels-Alder reaction with 2-alkenylthiopyranoquinoline-3-carbalde- hyde (derived from this compound) and various heterocyclic ketones [, ]. This method provides access to complex heterocyclic structures with high diastereoselectivity.
- Synthesis of iminothiophenone-fused quinolines: This reaction proceeds by reacting 2-Mercaptoquinoline-3-carbaldehydes with isocyanides in methanol, resulting in the formation of 2-(cyclohexylimino)thieno[2,3-b]quinolin-3(2H)-ones []. This method stands out for its atom economy and the formation of C–S and C–C bonds in a single step.
Q2: What are the advantages of using this compound in these reactions?
A2: Several factors contribute to the advantageous use of this compound in synthetic chemistry:
- One-pot synthesis: Many reactions utilizing this compound can be carried out in a one-pot fashion, simplifying the procedure and enhancing efficiency [, , ].
- Mild reaction conditions: Many of these reactions proceed under mild conditions, minimizing the formation of unwanted byproducts and increasing overall yield [].
- Potential for diversity-oriented synthesis: The diverse reactivity of this compound allows for the generation of a library of structurally diverse compounds, which is valuable for drug discovery and exploring novel chemical spaces [].
Q3: What types of catalysts are used in conjunction with this compound?
A3: The choice of catalyst depends on the specific reaction being performed. Examples include:
- L-proline: This naturally occurring amino acid has been employed as a catalyst in the three-component synthesis of 4H-substituted thiopyrano[2,3-b]quinolines derivatives [].
- Tetrabutylammonium hydrogensulfate: This phase-transfer catalyst has been utilized in the domino Knoevenagel/hetero-Diels-Alder reaction leading to thiochromenoquinoline-fused heterocycles [].
- Triphenylphosphine (TPP): This organophosphorus compound plays a crucial role in the synthesis of 2H-thiopyrano [2, 3-b] quinoline-2, 3-dicarboxylates, influencing the reaction mechanism and kinetics [, ].
Q4: Has the mechanism of any reactions involving this compound been investigated?
A4: Yes, the mechanism of the reaction between this compound, dialkyl acetylenedicarboxylates, and triphenylphosphine has been studied in detail []. The proposed mechanism involves six steps, including a fast initial step involving triphenylphosphine and the acetylenedicarboxylate. The rate-determining step is influenced by the structure of the dialkyl acetylenedicarboxylate used [].
Q5: What are the potential applications of the compounds synthesized using this compound?
A5: The synthesized compounds, especially the polycyclic heterocyclic structures, hold promise for various applications, including:
- Medicinal chemistry: Heterocyclic compounds are prevalent in pharmaceuticals, and these novel structures could exhibit biological activities relevant to drug discovery [, ].
Q6: What are some limitations or challenges associated with the use of this compound in synthesis?
A6: While a valuable building block, some limitations exist:
Q7: What are the future directions for research on this compound?
A7: Future research could focus on:
- Structure-activity relationship studies: Systematically modifying the structures of synthesized compounds and evaluating their biological activities will be crucial for identifying promising drug candidates and understanding the impact of structural modifications on activity [].
Q8: What analytical techniques are commonly used to characterize the compounds synthesized with this compound?
A8: Commonly employed analytical techniques include:
- Nuclear Magnetic Resonance (NMR) spectroscopy: This technique is crucial for determining the structure and stereochemistry of the synthesized compounds [, , ].
- Infrared (IR) spectroscopy: This method provides information about the functional groups present in the synthesized compounds [, ].
- Mass spectrometry: This technique determines the molecular weight and provides insights into the fragmentation pattern of the molecules, further supporting structural elucidation [, , ].
- X-ray crystallography: In some cases, obtaining single crystals of the products allows for the definitive determination of their three-dimensional structure [].
Disclaimer and Information on In-Vitro Research Products
Please be aware that all articles and product information presented on BenchChem are intended solely for informational purposes. The products available for purchase on BenchChem are specifically designed for in-vitro studies, which are conducted outside of living organisms. In-vitro studies, derived from the Latin term "in glass," involve experiments performed in controlled laboratory settings using cells or tissues. It is important to note that these products are not categorized as medicines or drugs, and they have not received approval from the FDA for the prevention, treatment, or cure of any medical condition, ailment, or disease. We must emphasize that any form of bodily introduction of these products into humans or animals is strictly prohibited by law. It is essential to adhere to these guidelines to ensure compliance with legal and ethical standards in research and experimentation.