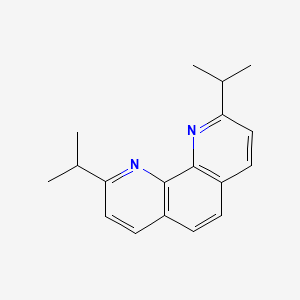
2,9-Diisopropyl-1,10-phenanthroline
- Click on QUICK INQUIRY to receive a quote from our team of experts.
- With the quality product at a COMPETITIVE price, you can focus more on your research.
Overview
Description
2,9-Diisopropyl-1,10-phenanthroline is an organic compound with the molecular formula C18H20N2. It is a derivative of 1,10-phenanthroline, where the hydrogen atoms at positions 2 and 9 are replaced by isopropyl groups. This modification imparts unique steric and electronic properties to the molecule, making it a valuable ligand in coordination chemistry and various scientific applications.
Preparation Methods
Synthetic Routes and Reaction Conditions
The synthesis of 2,9-Diisopropyl-1,10-phenanthroline typically involves the alkylation of 1,10-phenanthroline. One common method is the reaction of 1,10-phenanthroline with isopropyl bromide in the presence of a strong base such as potassium carbonate. The reaction is carried out in an aprotic solvent like dimethylformamide (DMF) at elevated temperatures to facilitate the substitution reaction.
Industrial Production Methods
While specific industrial production methods for this compound are not widely documented, the general approach would involve scaling up the laboratory synthesis. This would include optimizing reaction conditions, such as temperature, solvent, and reactant concentrations, to achieve high yields and purity. Industrial production may also involve continuous flow processes to enhance efficiency and scalability.
Chemical Reactions Analysis
Types of Reactions
2,9-Diisopropyl-1,10-phenanthroline undergoes various chemical reactions, including:
Coordination Reactions: It forms stable complexes with transition metals, such as copper(I), by coordinating through its nitrogen atoms.
Oxidation and Reduction: The compound can participate in redox reactions, particularly when coordinated to metal centers.
Substitution Reactions: The isopropyl groups can be substituted under specific conditions, although this is less common due to steric hindrance.
Common Reagents and Conditions
Coordination Reactions: Typically involve metal salts like copper(I) chloride or copper(I) bromide in solvents such as acetonitrile or dichloromethane.
Oxidation and Reduction: Common reagents include oxidizing agents like hydrogen peroxide or reducing agents like sodium borohydride.
Substitution Reactions: Require strong nucleophiles or electrophiles and may involve catalysts to overcome steric hindrance.
Major Products
Coordination Complexes: Metal-phenanthroline complexes, such as [Cu(this compound)2]+, which exhibit unique photophysical properties.
Oxidation Products: Oxidized derivatives of the phenanthroline ligand.
Substitution Products: Substituted phenanthroline derivatives, though less common.
Scientific Research Applications
2,9-Diisopropyl-1,10-phenanthroline has a wide range of applications in scientific research:
Chemistry: Used as a ligand in coordination chemistry to form stable metal complexes with unique electronic and photophysical properties.
Biology: Employed in studies involving metal ion detection and quantification due to its strong binding affinity for transition metals.
Medicine: Investigated for potential therapeutic applications, such as in the development of metal-based drugs and diagnostic agents.
Industry: Utilized in catalysis, particularly in processes involving redox reactions and photochemical transformations.
Mechanism of Action
The mechanism of action of 2,9-Diisopropyl-1,10-phenanthroline primarily involves its ability to coordinate with metal ions through its nitrogen atoms. This coordination can stabilize metal centers, facilitate electron transfer processes, and enhance the photophysical properties of the resulting complexes. The steric bulk of the isopropyl groups also influences the geometry and stability of the metal complexes, impacting their reactivity and applications.
Comparison with Similar Compounds
Similar Compounds
2,9-Dimethyl-1,10-phenanthroline: Similar in structure but with methyl groups instead of isopropyl groups, leading to different steric and electronic properties.
2,9-Di-tert-butyl-1,10-phenanthroline: Features larger tert-butyl groups, resulting in greater steric hindrance and different coordination behavior.
1,10-Phenanthroline: The parent compound without any substituents at positions 2 and 9, offering less steric hindrance and different reactivity.
Uniqueness
2,9-Diisopropyl-1,10-phenanthroline is unique due to the balance it offers between steric bulk and electronic properties. The isopropyl groups provide sufficient steric hindrance to influence the coordination environment without being overly bulky, making it a versatile ligand in various applications.
Properties
IUPAC Name |
2,9-di(propan-2-yl)-1,10-phenanthroline |
Source
|
---|---|---|
Details | Computed by Lexichem TK 2.7.0 (PubChem release 2021.05.07) | |
Source | PubChem | |
URL | https://pubchem.ncbi.nlm.nih.gov | |
Description | Data deposited in or computed by PubChem | |
InChI |
InChI=1S/C18H20N2/c1-11(2)15-9-7-13-5-6-14-8-10-16(12(3)4)20-18(14)17(13)19-15/h5-12H,1-4H3 |
Source
|
Details | Computed by InChI 1.0.6 (PubChem release 2021.05.07) | |
Source | PubChem | |
URL | https://pubchem.ncbi.nlm.nih.gov | |
Description | Data deposited in or computed by PubChem | |
InChI Key |
RADRKVQODOBYPB-UHFFFAOYSA-N |
Source
|
Details | Computed by InChI 1.0.6 (PubChem release 2021.05.07) | |
Source | PubChem | |
URL | https://pubchem.ncbi.nlm.nih.gov | |
Description | Data deposited in or computed by PubChem | |
Canonical SMILES |
CC(C)C1=NC2=C(C=CC3=C2N=C(C=C3)C(C)C)C=C1 |
Source
|
Details | Computed by OEChem 2.3.0 (PubChem release 2021.05.07) | |
Source | PubChem | |
URL | https://pubchem.ncbi.nlm.nih.gov | |
Description | Data deposited in or computed by PubChem | |
Molecular Formula |
C18H20N2 |
Source
|
Details | Computed by PubChem 2.1 (PubChem release 2021.05.07) | |
Source | PubChem | |
URL | https://pubchem.ncbi.nlm.nih.gov | |
Description | Data deposited in or computed by PubChem | |
DSSTOX Substance ID |
DTXSID10573634 |
Source
|
Record name | 2,9-Di(propan-2-yl)-1,10-phenanthroline | |
Source | EPA DSSTox | |
URL | https://comptox.epa.gov/dashboard/DTXSID10573634 | |
Description | DSSTox provides a high quality public chemistry resource for supporting improved predictive toxicology. | |
Molecular Weight |
264.4 g/mol |
Source
|
Details | Computed by PubChem 2.1 (PubChem release 2021.05.07) | |
Source | PubChem | |
URL | https://pubchem.ncbi.nlm.nih.gov | |
Description | Data deposited in or computed by PubChem | |
CAS No. |
869900-81-2 |
Source
|
Record name | 2,9-Di(propan-2-yl)-1,10-phenanthroline | |
Source | EPA DSSTox | |
URL | https://comptox.epa.gov/dashboard/DTXSID10573634 | |
Description | DSSTox provides a high quality public chemistry resource for supporting improved predictive toxicology. | |
Disclaimer and Information on In-Vitro Research Products
Please be aware that all articles and product information presented on BenchChem are intended solely for informational purposes. The products available for purchase on BenchChem are specifically designed for in-vitro studies, which are conducted outside of living organisms. In-vitro studies, derived from the Latin term "in glass," involve experiments performed in controlled laboratory settings using cells or tissues. It is important to note that these products are not categorized as medicines or drugs, and they have not received approval from the FDA for the prevention, treatment, or cure of any medical condition, ailment, or disease. We must emphasize that any form of bodily introduction of these products into humans or animals is strictly prohibited by law. It is essential to adhere to these guidelines to ensure compliance with legal and ethical standards in research and experimentation.