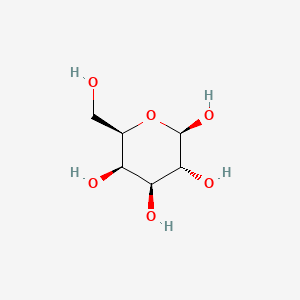
beta-D-galactose
Overview
Description
Beta-D-galactose is a D-galactopyranose having beta-configuration at the anomeric centre. It has a role as an epitope and a mouse metabolite. It is an enantiomer of a beta-L-galactose.
This compound is a metabolite found in or produced by Escherichia coli (strain K12, MG1655).
This compound contains a Galactosylceramide motif and is often attached to a Cer aglycon.
This compound is a natural product found in Symphytum tuberosum with data available.
Mechanism of Action
Target of Action
Beta-D-Galactose is a simple natural compound that has been investigated for its distinctive properties and interactions with specific cell receptors . It functions as a ligand to selectively target cells expressing galactose receptors, such as hepatocytes, macrophages, and specific cancer cells . The direct attachment of galactose to the main drug or to drug-loaded nanoparticles or liposomes enhances cellular uptake, thereby improving drug delivery to the intended target cells .
Mode of Action
This compound, also known as lactase, is an exo-glycosidase enzyme that hydrolyzes the β-glycosidic bond present between a terminal galactose residue and its organic moiety . This enzyme catalyzes the transfer of a sugar residue from a glycosyl donor to an acceptor via a double-displacement mechanism . Hydrolysis prevails when water acts as an acceptor, resulting in the production of lactose-free products .
Biochemical Pathways
This compound is primarily metabolized via the Leloir pathway in the liver . This pathway is highly conserved and consists of four enzymes: galactose mutarotase (GALM, EC 5.1.3.3), galactokinase (GALK1, EC 2.7.1.6), galactose-1-phosphate uridylyltransferase (GALT, EC 2.7.7.12), and UDP-galactose-4’-epimerase (GALE, EC 5.1.3.2) . The conversion of this compound to glucose 1-phosphate is accomplished by the action of these four enzymes .
Pharmacokinetics
The pharmacokinetics of this compound are influenced by its use in drug delivery systems. For instance, when attached to the main drug or to drug-loaded nanoparticles or liposomes, it enhances cellular uptake, thereby improving drug delivery to the intended target cells . This results in improved bioavailability of the drug.
Result of Action
The hydrolysis of lactose to glucose and galactose by Beta-D-Galactosidase is an important process in the food industry because of the potentially beneficial effects of assimilating food containing lactose . In addition, the enzyme catalyzes the transgalactosylation process by transferring the galactose molecule to a different nucleophile containing a hydroxyl group . This transgalactosylation process results in the production of industrially valuable prebiotic oligosaccharides .
Action Environment
The action of this compound is influenced by various environmental factors. For instance, the structural conditions of the supports used in enzyme immobilization are determining factors in the performance of the biocatalysts . Furthermore, the presence of specific active groups in the support allows the generation of interactions . These factors can influence the compound’s action, efficacy, and stability.
Biochemical Analysis
Biochemical Properties
Beta-D-Galactose interacts with several enzymes, proteins, and other biomolecules. One of the most notable enzymes it interacts with is beta-galactosidase, a glycoside hydrolase enzyme. This enzyme catalyzes the hydrolysis of the glycosidic bond in lactose, splitting it into glucose and galactose . The interaction between this compound and beta-galactosidase is a key biochemical reaction that enables lactose digestion .
Cellular Effects
This compound has significant effects on various types of cells and cellular processes. It influences cell function by participating in metabolic pathways that provide energy for the cells. It also impacts gene expression and cellular metabolism, as it is a fundamental building block for the synthesis of other biomolecules .
Molecular Mechanism
The molecular mechanism of action of this compound involves its interaction with enzymes like beta-galactosidase. This enzyme catalyzes the hydrolysis of lactose into glucose and galactose, a reaction that is essential for lactose digestion . This compound can also bind to other biomolecules, influencing their function and activity .
Temporal Effects in Laboratory Settings
In laboratory settings, the effects of this compound can change over time. Its stability and degradation are important factors to consider in these settings. Long-term effects on cellular function have been observed in in vitro or in vivo studies .
Dosage Effects in Animal Models
The effects of this compound can vary with different dosages in animal models. High doses of this compound have been used in research to induce aging-like changes in animals, providing a model for studying aging and age-related diseases .
Metabolic Pathways
This compound is involved in several metabolic pathways. It is a key player in the Leloir pathway, a metabolic pathway responsible for the conversion of galactose to glucose .
Transport and Distribution
This compound is transported and distributed within cells and tissues through various mechanisms. It can be transported across cell membranes by specific transport proteins .
Subcellular Localization
This compound, as a fundamental monosaccharide, does not have a specific subcellular localization. The enzymes that metabolize this compound, such as beta-galactosidase, are typically localized in the cytoplasm .
Properties
IUPAC Name |
(2R,3R,4S,5R,6R)-6-(hydroxymethyl)oxane-2,3,4,5-tetrol | |
---|---|---|
Source | PubChem | |
URL | https://pubchem.ncbi.nlm.nih.gov | |
Description | Data deposited in or computed by PubChem | |
InChI |
InChI=1S/C6H12O6/c7-1-2-3(8)4(9)5(10)6(11)12-2/h2-11H,1H2/t2-,3+,4+,5-,6-/m1/s1 | |
Source | PubChem | |
URL | https://pubchem.ncbi.nlm.nih.gov | |
Description | Data deposited in or computed by PubChem | |
InChI Key |
WQZGKKKJIJFFOK-FPRJBGLDSA-N | |
Source | PubChem | |
URL | https://pubchem.ncbi.nlm.nih.gov | |
Description | Data deposited in or computed by PubChem | |
Canonical SMILES |
C(C1C(C(C(C(O1)O)O)O)O)O | |
Source | PubChem | |
URL | https://pubchem.ncbi.nlm.nih.gov | |
Description | Data deposited in or computed by PubChem | |
Isomeric SMILES |
C([C@@H]1[C@@H]([C@@H]([C@H]([C@@H](O1)O)O)O)O)O | |
Source | PubChem | |
URL | https://pubchem.ncbi.nlm.nih.gov | |
Description | Data deposited in or computed by PubChem | |
Molecular Formula |
C6H12O6 | |
Source | PubChem | |
URL | https://pubchem.ncbi.nlm.nih.gov | |
Description | Data deposited in or computed by PubChem | |
DSSTOX Substance ID |
DTXSID001015884 | |
Record name | beta-D-Galactopyranose | |
Source | EPA DSSTox | |
URL | https://comptox.epa.gov/dashboard/DTXSID001015884 | |
Description | DSSTox provides a high quality public chemistry resource for supporting improved predictive toxicology. | |
Molecular Weight |
180.16 g/mol | |
Source | PubChem | |
URL | https://pubchem.ncbi.nlm.nih.gov | |
Description | Data deposited in or computed by PubChem | |
Physical Description |
Solid | |
Record name | beta-D-Galactose | |
Source | Human Metabolome Database (HMDB) | |
URL | http://www.hmdb.ca/metabolites/HMDB0003449 | |
Description | The Human Metabolome Database (HMDB) is a freely available electronic database containing detailed information about small molecule metabolites found in the human body. | |
Explanation | HMDB is offered to the public as a freely available resource. Use and re-distribution of the data, in whole or in part, for commercial purposes requires explicit permission of the authors and explicit acknowledgment of the source material (HMDB) and the original publication (see the HMDB citing page). We ask that users who download significant portions of the database cite the HMDB paper in any resulting publications. | |
CAS No. |
7296-64-2 | |
Record name | β-D-Galactopyranose | |
Source | CAS Common Chemistry | |
URL | https://commonchemistry.cas.org/detail?cas_rn=7296-64-2 | |
Description | CAS Common Chemistry is an open community resource for accessing chemical information. Nearly 500,000 chemical substances from CAS REGISTRY cover areas of community interest, including common and frequently regulated chemicals, and those relevant to high school and undergraduate chemistry classes. This chemical information, curated by our expert scientists, is provided in alignment with our mission as a division of the American Chemical Society. | |
Explanation | The data from CAS Common Chemistry is provided under a CC-BY-NC 4.0 license, unless otherwise stated. | |
Record name | beta-D-Galactose | |
Source | ChemIDplus | |
URL | https://pubchem.ncbi.nlm.nih.gov/substance/?source=chemidplus&sourceid=0007296642 | |
Description | ChemIDplus is a free, web search system that provides access to the structure and nomenclature authority files used for the identification of chemical substances cited in National Library of Medicine (NLM) databases, including the TOXNET system. | |
Record name | beta-D-Galactopyranose | |
Source | EPA DSSTox | |
URL | https://comptox.epa.gov/dashboard/DTXSID001015884 | |
Description | DSSTox provides a high quality public chemistry resource for supporting improved predictive toxicology. | |
Record name | β-D-Galactopyranose | |
Source | FDA Global Substance Registration System (GSRS) | |
URL | https://gsrs.ncats.nih.gov/ginas/app/beta/substances/RQ6K52J67A | |
Description | The FDA Global Substance Registration System (GSRS) enables the efficient and accurate exchange of information on what substances are in regulated products. Instead of relying on names, which vary across regulatory domains, countries, and regions, the GSRS knowledge base makes it possible for substances to be defined by standardized, scientific descriptions. | |
Explanation | Unless otherwise noted, the contents of the FDA website (www.fda.gov), both text and graphics, are not copyrighted. They are in the public domain and may be republished, reprinted and otherwise used freely by anyone without the need to obtain permission from FDA. Credit to the U.S. Food and Drug Administration as the source is appreciated but not required. | |
Record name | beta-D-Galactose | |
Source | Human Metabolome Database (HMDB) | |
URL | http://www.hmdb.ca/metabolites/HMDB0003449 | |
Description | The Human Metabolome Database (HMDB) is a freely available electronic database containing detailed information about small molecule metabolites found in the human body. | |
Explanation | HMDB is offered to the public as a freely available resource. Use and re-distribution of the data, in whole or in part, for commercial purposes requires explicit permission of the authors and explicit acknowledgment of the source material (HMDB) and the original publication (see the HMDB citing page). We ask that users who download significant portions of the database cite the HMDB paper in any resulting publications. | |
Retrosynthesis Analysis
AI-Powered Synthesis Planning: Our tool employs the Template_relevance Pistachio, Template_relevance Bkms_metabolic, Template_relevance Pistachio_ringbreaker, Template_relevance Reaxys, Template_relevance Reaxys_biocatalysis model, leveraging a vast database of chemical reactions to predict feasible synthetic routes.
One-Step Synthesis Focus: Specifically designed for one-step synthesis, it provides concise and direct routes for your target compounds, streamlining the synthesis process.
Accurate Predictions: Utilizing the extensive PISTACHIO, BKMS_METABOLIC, PISTACHIO_RINGBREAKER, REAXYS, REAXYS_BIOCATALYSIS database, our tool offers high-accuracy predictions, reflecting the latest in chemical research and data.
Strategy Settings
Precursor scoring | Relevance Heuristic |
---|---|
Min. plausibility | 0.01 |
Model | Template_relevance |
Template Set | Pistachio/Bkms_metabolic/Pistachio_ringbreaker/Reaxys/Reaxys_biocatalysis |
Top-N result to add to graph | 6 |
Feasible Synthetic Routes
Q1: How does β-D-galactose interact with biological systems?
A1: β-D-galactose plays a crucial role in various biological processes. It interacts with specific proteins called lectins. For instance, Peanut (Arachis hypogaea) agglutinin (PNA) exhibits a strong binding affinity for β-D-galactose. [] This interaction is highly specific, with PNA showing a preference for β-D-galactose over glucose analogues. []
Q2: Can you elaborate on the implications of β-D-galactose's interaction with antiganglioside antibodies?
A2: Research indicates that a significant proportion of antiganglioside antibodies, key components of the innate immune system, also bind to 3-O-sulfo-β-D-galactosylceramide (sulfatide). [] This interaction suggests a potential link between antiganglioside antibodies and sulfatide, a molecule abundant in plasma and peripheral nerve myelin. [] This finding might offer insights into the immunoregulatory roles of antiganglioside antibodies. Notably, the spatial presentation of sulfatide significantly influences antibody binding, being absent when integrated into membranes like myelin. [] This suggests a context-dependent interaction with potential functional implications.
Q3: What is the significance of β-D-galactose in the context of apoptosis?
A3: Studies have revealed a connection between β-D-galactose and apoptosis. Specifically, an increased expression of plasma membrane glycoproteins enriched in α-D-mannose and β-D-galactose was observed in apoptotic murine leukemia L1210 cells. [] This heightened expression was consistent across various cell lines, tissue origins, and apoptosis-inducing agents. [] This finding suggests a potential role for these glycoproteins as general markers for detecting apoptotic cells.
Q4: What is the molecular structure of β-D-galactose?
A4: β-D-galactose is a six-carbon sugar (hexose) and an epimer of glucose, differing only in the orientation of the hydroxyl group at the C4 position. It exists predominantly as a pyranose ring in solution.
Q5: Is there any spectroscopic data available for the binding interaction of β-D-galactose?
A5: Yes, proton NMR spectroscopy studies have provided valuable insights into how β-D-galactose interacts with proteins. For example, research investigated the binding of methyl-β-D-galactoses to sialic acid residues on Hepatic Binding Protein (HBP). [] The study revealed that calcium ions (Ca2+) play a role in stabilizing the binding interaction between these molecules. [] This observation highlights the influence of metal ions on carbohydrate-protein interactions, which is crucial for understanding biological recognition processes.
Q6: How is β-D-galactose involved in enzyme activity?
A6: β-D-galactose serves as a substrate for various enzymes, particularly those belonging to the glycosidase family. For instance, β-D-galactosidase, an enzyme found in many organisms, catalyzes the hydrolysis of β-D-galactosidic linkages in various substrates, including lactose. This enzyme plays a vital role in lactose metabolism and has significant biotechnological applications.
Q7: Are there any studies on the inhibition of enzymes that utilize β-D-galactose?
A7: Yes, research has investigated the inhibitory effects of various molecules on enzymes that utilize β-D-galactose. A study focusing on insulin release from pancreatic islets revealed that both α-D-galactose pentaacetate and β-D-galactose pentaacetate inhibit leucine-induced insulin release. [] This inhibition suggests a potential role for D-galactose pentaacetate esters in regulating insulin secretion and highlights a potential therapeutic avenue for managing conditions like hyperinsulinemia.
Q8: How is computational chemistry being used to understand β-D-galactose?
A8: Computational methods provide valuable insights into the conformational properties of β-D-galactose. For example, a study combined ab initio electronic energy calculations and torsional path integral Monte Carlo simulations to assess the impact of torsional anharmonicity on the molecule's conformational behavior. [] The research revealed that while the global minimum structure dominates at low temperatures (100 K), a diverse range of conformers populate at ambient and higher temperatures. [] These findings enhance our understanding of β-D-galactose's flexibility and its potential to adopt various conformations in different biological contexts.
Q9: Are there any specific drug delivery strategies being explored using β-D-galactose?
A9: Research suggests that the targeted delivery of therapeutic agents can be achieved by exploiting the binding specificity of β-D-galactose to specific receptors. One such example is the use of galactosylated human serum albumin (GSA) in NIR-PIT. [] By conjugating GSA with a photo-absorber, researchers successfully targeted β-D-galactose receptors overexpressed on cancer cells. [] This targeted approach enhances the therapeutic index by concentrating the cytotoxic effects at the tumor site while minimizing off-target toxicity.
Q10: How can β-D-galactose be utilized in diagnostic applications?
A10: Research indicates that β-D-galactose can be exploited as a target for diagnostic tools. For instance, the enzymatic activity of β-D-galactosidase can be used to detect and quantify lactose. [] This is particularly relevant in the food industry for monitoring lactose levels in dairy products.
Disclaimer and Information on In-Vitro Research Products
Please be aware that all articles and product information presented on BenchChem are intended solely for informational purposes. The products available for purchase on BenchChem are specifically designed for in-vitro studies, which are conducted outside of living organisms. In-vitro studies, derived from the Latin term "in glass," involve experiments performed in controlled laboratory settings using cells or tissues. It is important to note that these products are not categorized as medicines or drugs, and they have not received approval from the FDA for the prevention, treatment, or cure of any medical condition, ailment, or disease. We must emphasize that any form of bodily introduction of these products into humans or animals is strictly prohibited by law. It is essential to adhere to these guidelines to ensure compliance with legal and ethical standards in research and experimentation.