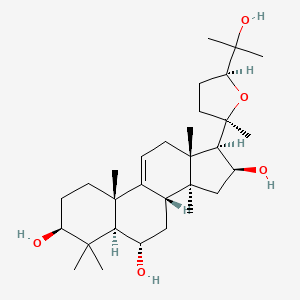
Astragenol
Overview
Description
Mechanism of Action
Target of Action
This compound, also known as Cyclothis compound (CAG), is a tetracyclic triterpenoid isolated from the Astragalus genus . It is known to primarily target the human enzyme telomerase . Telomerase is an enzyme that adds DNA sequence repeats to the 3’ end of DNA strands in the telomere regions, which are found at the ends of chromosomes . This action of telomerase helps prevent the loss of important DNA from chromosome ends, thereby protecting the genetic data and enabling cells to divide correctly .
Mode of Action
This compound interacts with its target, telomerase, by activating it . This activation leads to an increase in telomerase activity, which may inhibit the onset of cellular senescence . Cellular senescence is a state in which normal healthy cells lose the ability to divide. By delaying this process, this compound could potentially extend the lifespan of cells .
Biochemical Pathways
This compound affects several biochemical pathways. One key pathway is the MAP kinase pathway . This compound has been shown to downregulate the expression of phospho c-Jun-N-terminal kinase (p-JNK), p-38, and phospho-extracellular signal-related kinase (ERK1/2) in the brains of mice injected with Amyloid-beta (Aβ), a peptide linked to Alzheimer’s disease . This suggests that this compound may have a neuroprotective effect .
Pharmacokinetics
It is known that this compound is more lipid-soluble and more easily absorbed transdermally compared to astragaloside iv (asi), from which it is derived . Encapsulation of this compound in phospholipid vesicles has been shown to enhance its transport and delivery across the skin barrier , suggesting a potential method for improving its bioavailability.
Result of Action
The molecular and cellular effects of this compound’s action are diverse. It has been shown to regulate oxidative stress, neurotrophic dysfunctions, neuroinflammation, and apoptotic cell death . For instance, this compound has been found to elevate the expression of Nrf2 (nuclear factor erythroid 2–related factor 2), HO-1 (heme oxygenase-1), p-TrKB, BDNF, and NeuN in the brains of mice co-treated with Aβ and this compound . These findings suggest that this compound may have neuroprotective effects and could potentially be used in the treatment of neurodegenerative conditions .
Biochemical Analysis
Biochemical Properties
Astragenol interacts with various enzymes, proteins, and other biomolecules. It is involved in the regulation of oxidative stress, neurogenic dysfunction, activated mitogen-activated protein (MAP) kinases, and mitochondrial apoptosis . The compound this compound is also known to moderately increase telomerase activity and inhibit the onset of cellular senescence .
Cellular Effects
This compound has shown significant effects on various types of cells and cellular processes. It influences cell function by regulating oxidative stress, neurogenic dysfunction, neuroinflammation, and apoptotic cell death . This compound also impacts cell signaling pathways, gene expression, and cellular metabolism .
Molecular Mechanism
This compound exerts its effects at the molecular level through various mechanisms. It has been found to regulate oxidative stress, neurotrophic dysfunctions, neuroinflammation, and apoptotic cell death in an Aβ-induced mouse model of Alzheimer’s disease (AD) . This compound also has binding interactions with biomolecules, enzyme inhibition or activation, and changes in gene expression .
Temporal Effects in Laboratory Settings
The effects of this compound change over time in laboratory settings. Studies have shown that this compound regulates oxidative stress, neurotrophic processes, neuroinflammation, apoptotic cell death, and memory impairment in the mouse model of AD .
Dosage Effects in Animal Models
The effects of this compound vary with different dosages in animal models. While specific dosage effects are still being studied, this compound has been shown to have pro-cell proliferative activity and promote wound closure by activating telomerase .
Metabolic Pathways
This compound is involved in various metabolic pathways. The biosynthetic pathway of astragalosides in plants includes the mevalonate (MVA) pathway and the 2-C-methl-D-erythritol-4-phospate (MEP) pathway . These pathways ultimately produce the precursor isopentenyl pyrophosphate (IPP) .
Transport and Distribution
This compound is transported and distributed within cells and tissues. It has been shown that encapsulation of this compound in phospholipid vesicles enhances the transport and delivery across the skin barrier , which can be a new practical approach to make it more bioavailable for transdermal absorption.
Preparation Methods
Synthetic Routes and Reaction Conditions
Astragenol can be synthesized through the hydrolysis of astragaloside IV, a glycoside found in Astragalus membranaceus. There are three primary methods for this hydrolysis:
Acid Hydrolysis: This method involves using acidic conditions to break the glycosidic bonds in astragaloside IV, resulting in the formation of this compound.
Enzymatic Hydrolysis: Specific enzymes, such as β-xylosidase and β-glucosidase, are used to catalyze the hydrolysis of astragaloside IV into this compound.
Smith Degradation: This method involves the oxidative cleavage of glycosidic bonds, followed by reduction and hydrolysis steps to yield this compound.
Industrial Production Methods
Industrial production of this compound typically involves the enzymatic hydrolysis method due to its higher efficiency and specificity. The use of recombinant glycosidases has been shown to significantly improve the yield of this compound in industrial settings .
Chemical Reactions Analysis
Types of Reactions
Astragenol undergoes various chemical reactions, including:
Oxidation: This compound can be oxidized to form different derivatives, such as 20(27)-octanor-cyclothis compound.
Substitution: Substitution reactions can introduce new functional groups into the this compound molecule, altering its chemical properties.
Common Reagents and Conditions
Oxidation: Jones reagent is commonly used for the oxidation of this compound.
Reduction: Various reducing agents, such as sodium borohydride, can be used for the reduction of this compound.
Substitution: Different nucleophiles can be used in substitution reactions to introduce new functional groups into the this compound molecule.
Major Products Formed
The major products formed from these reactions include various oxidized, reduced, and substituted derivatives of this compound, each with unique chemical and biological properties .
Scientific Research Applications
Astragenol has a wide range of scientific research applications, including:
Comparison with Similar Compounds
Astragenol is structurally similar to other triterpenoid saponins isolated from the Astragalus genus, such as astragaloside IV, astragaloside I, and astragaloside II . this compound is unique in its higher lipid solubility and better transdermal absorption compared to its glycoside counterparts . This makes it more bioavailable and effective in various therapeutic applications.
List of Similar Compounds
- Astragaloside IV
- Astragaloside I
- Astragaloside II
- Astrasieversianin VII
- Isoastragaloside I
- Astragaloside III
This compound’s unique properties and wide range of applications make it a compound of significant interest in both scientific research and industrial applications.
Properties
IUPAC Name |
(3S,5R,6S,8S,10S,13R,14S,16S,17R)-17-[(2R,5S)-5-(2-hydroxypropan-2-yl)-2-methyloxolan-2-yl]-4,4,10,13,14-pentamethyl-2,3,5,6,7,8,12,15,16,17-decahydro-1H-cyclopenta[a]phenanthrene-3,6,16-triol | |
---|---|---|
Details | Computed by Lexichem TK 2.7.0 (PubChem release 2021.05.07) | |
Source | PubChem | |
URL | https://pubchem.ncbi.nlm.nih.gov | |
Description | Data deposited in or computed by PubChem | |
InChI |
InChI=1S/C30H50O5/c1-25(2)21(33)10-12-27(5)17-9-13-28(6)24(30(8)14-11-22(35-30)26(3,4)34)20(32)16-29(28,7)18(17)15-19(31)23(25)27/h9,18-24,31-34H,10-16H2,1-8H3/t18-,19+,20+,21+,22+,23+,24+,27-,28-,29+,30-/m1/s1 | |
Details | Computed by InChI 1.0.6 (PubChem release 2021.05.07) | |
Source | PubChem | |
URL | https://pubchem.ncbi.nlm.nih.gov | |
Description | Data deposited in or computed by PubChem | |
InChI Key |
KNESISUQBYQIIU-LOIFQKOGSA-N | |
Details | Computed by InChI 1.0.6 (PubChem release 2021.05.07) | |
Source | PubChem | |
URL | https://pubchem.ncbi.nlm.nih.gov | |
Description | Data deposited in or computed by PubChem | |
Canonical SMILES |
CC1(C(CCC2(C1C(CC3C2=CCC4(C3(CC(C4C5(CCC(O5)C(C)(C)O)C)O)C)C)O)C)O)C | |
Details | Computed by OEChem 2.3.0 (PubChem release 2021.05.07) | |
Source | PubChem | |
URL | https://pubchem.ncbi.nlm.nih.gov | |
Description | Data deposited in or computed by PubChem | |
Isomeric SMILES |
C[C@@]1(CC[C@H](O1)C(C)(C)O)[C@H]2[C@H](C[C@@]3([C@@]2(CC=C4[C@H]3C[C@@H]([C@@H]5[C@@]4(CC[C@@H](C5(C)C)O)C)O)C)C)O | |
Details | Computed by OEChem 2.3.0 (PubChem release 2021.05.07) | |
Source | PubChem | |
URL | https://pubchem.ncbi.nlm.nih.gov | |
Description | Data deposited in or computed by PubChem | |
Molecular Formula |
C30H50O5 | |
Details | Computed by PubChem 2.1 (PubChem release 2021.05.07) | |
Source | PubChem | |
URL | https://pubchem.ncbi.nlm.nih.gov | |
Description | Data deposited in or computed by PubChem | |
DSSTOX Substance ID |
DTXSID401170200 | |
Record name | (3β,6α,16β,20R,24S)-20,24-Epoxylanost-9(11)-ene-3,6,16,25-tetrol | |
Source | EPA DSSTox | |
URL | https://comptox.epa.gov/dashboard/DTXSID401170200 | |
Description | DSSTox provides a high quality public chemistry resource for supporting improved predictive toxicology. | |
Molecular Weight |
490.7 g/mol | |
Details | Computed by PubChem 2.1 (PubChem release 2021.05.07) | |
Source | PubChem | |
URL | https://pubchem.ncbi.nlm.nih.gov | |
Description | Data deposited in or computed by PubChem | |
CAS No. |
86541-79-9 | |
Record name | (3β,6α,16β,20R,24S)-20,24-Epoxylanost-9(11)-ene-3,6,16,25-tetrol | |
Source | CAS Common Chemistry | |
URL | https://commonchemistry.cas.org/detail?cas_rn=86541-79-9 | |
Description | CAS Common Chemistry is an open community resource for accessing chemical information. Nearly 500,000 chemical substances from CAS REGISTRY cover areas of community interest, including common and frequently regulated chemicals, and those relevant to high school and undergraduate chemistry classes. This chemical information, curated by our expert scientists, is provided in alignment with our mission as a division of the American Chemical Society. | |
Explanation | The data from CAS Common Chemistry is provided under a CC-BY-NC 4.0 license, unless otherwise stated. | |
Record name | (3β,6α,16β,20R,24S)-20,24-Epoxylanost-9(11)-ene-3,6,16,25-tetrol | |
Source | EPA DSSTox | |
URL | https://comptox.epa.gov/dashboard/DTXSID401170200 | |
Description | DSSTox provides a high quality public chemistry resource for supporting improved predictive toxicology. | |
Retrosynthesis Analysis
AI-Powered Synthesis Planning: Our tool employs the Template_relevance Pistachio, Template_relevance Bkms_metabolic, Template_relevance Pistachio_ringbreaker, Template_relevance Reaxys, Template_relevance Reaxys_biocatalysis model, leveraging a vast database of chemical reactions to predict feasible synthetic routes.
One-Step Synthesis Focus: Specifically designed for one-step synthesis, it provides concise and direct routes for your target compounds, streamlining the synthesis process.
Accurate Predictions: Utilizing the extensive PISTACHIO, BKMS_METABOLIC, PISTACHIO_RINGBREAKER, REAXYS, REAXYS_BIOCATALYSIS database, our tool offers high-accuracy predictions, reflecting the latest in chemical research and data.
Strategy Settings
Precursor scoring | Relevance Heuristic |
---|---|
Min. plausibility | 0.01 |
Model | Template_relevance |
Template Set | Pistachio/Bkms_metabolic/Pistachio_ringbreaker/Reaxys/Reaxys_biocatalysis |
Top-N result to add to graph | 6 |
Feasible Synthetic Routes
Disclaimer and Information on In-Vitro Research Products
Please be aware that all articles and product information presented on BenchChem are intended solely for informational purposes. The products available for purchase on BenchChem are specifically designed for in-vitro studies, which are conducted outside of living organisms. In-vitro studies, derived from the Latin term "in glass," involve experiments performed in controlled laboratory settings using cells or tissues. It is important to note that these products are not categorized as medicines or drugs, and they have not received approval from the FDA for the prevention, treatment, or cure of any medical condition, ailment, or disease. We must emphasize that any form of bodily introduction of these products into humans or animals is strictly prohibited by law. It is essential to adhere to these guidelines to ensure compliance with legal and ethical standards in research and experimentation.