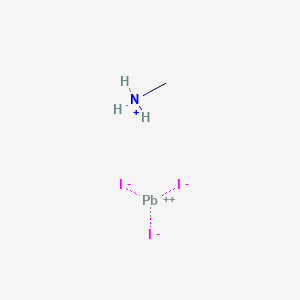
Perovskite CH3NH3PbI3 Powder
Overview
Description
Perovskite CH3NH3PbI3 Powder, also known as methylammonium lead iodide, is an organic-inorganic hybrid material that has gained significant attention in recent years. This compound is part of the perovskite family, which is known for its unique crystal structure and remarkable optoelectronic properties. Perovskite CH3NH3PbI3 has shown great potential in various applications, particularly in the field of solar cells, due to its high power conversion efficiency and ease of fabrication .
Preparation Methods
Synthetic Routes and Reaction Conditions
The synthesis of Perovskite CH3NH3PbI3 typically involves the reaction of methylammonium iodide (CH3NH3I) with lead iodide (PbI2) in a solvent such as gamma-butyrolactone or dimethylformamide. One common method is the solution-based approach, where the precursors are dissolved in the solvent and then deposited onto a substrate via spin-coating or other techniques. The film is then annealed to form the perovskite structure .
Industrial Production Methods
In industrial settings, the production of Perovskite CH3NH3PbI3 can be scaled up using techniques such as vapor deposition or mechanosynthesis. Mechanosynthesis involves grinding the precursors together in a ball mill, which can produce highly crystalline perovskite particles with excellent purity and stability .
Chemical Reactions Analysis
Types of Reactions
Perovskite CH3NH3PbI3 undergoes various chemical reactions, including oxidation, reduction, and substitution. These reactions can significantly impact the material’s properties and stability.
Common Reagents and Conditions
Major Products Formed
The major products formed from these reactions include lead oxide, mixed-halide perovskites, and various degradation products depending on the specific reaction conditions .
Scientific Research Applications
Perovskite CH3NH3PbI3 has a wide range of scientific research applications due to its unique properties:
Solar Cells: It is widely used in the fabrication of high-efficiency perovskite solar cells, with power conversion efficiencies exceeding 20%
Light-Emitting Devices: Perovskite CH3NH3PbI3 is also explored for use in light-emitting diodes (LEDs) due to its excellent luminescent properties.
Photodetectors: Its high absorption coefficient and charge carrier mobility make it an ideal candidate for photodetector applications.
Mechanism of Action
The mechanism by which Perovskite CH3NH3PbI3 exerts its effects is primarily related to its ability to absorb light and generate charge carriers (electrons and holes). When exposed to light, the material absorbs photons, creating electron-hole pairs. These charge carriers are then transported through the perovskite layer to the respective electrodes, generating an electric current. The efficiency of this process is influenced by the material’s crystal structure, bandgap, and charge carrier mobility .
Comparison with Similar Compounds
Perovskite CH3NH3PbI3 can be compared with other similar compounds such as:
CH3NH3PbCl3: This compound has a similar structure but different halide ions, resulting in different optical and electronic properties.
CH3NH3PbBr3: Another halide variant, which offers different bandgap and stability characteristics.
CsSnI3: A lead-free perovskite material that has gained attention for its potential environmental benefits.
CH3NH3GeCl3: A germanium-based perovskite that offers different electronic properties compared to lead-based perovskites.
Perovskite CH3NH3PbI3 stands out due to its optimal bandgap for solar cell applications, high absorption coefficient, and ease of fabrication, making it a preferred choice for many researchers .
Biological Activity
Methylammonium lead triiodide (CH3NH3PbI3), commonly known as MAPbI3, is a hybrid organic-inorganic perovskite that has garnered significant attention in the fields of photovoltaics and materials science. While its primary applications have been in solar energy conversion, recent studies have begun to explore its biological activity, particularly in the context of toxicity and environmental impact.
Overview of Biological Activity
The biological activity of MAPbI3 primarily revolves around its interactions with biological systems, particularly due to the presence of lead, which is known for its toxicological effects. The compound's stability and degradation pathways in biological environments are critical areas of research, given their implications for environmental health and safety.
Key Findings
- Toxicity of Lead : Lead is a well-documented neurotoxin, and its presence in MAPbI3 raises concerns regarding the compound's safety in applications that may lead to environmental exposure. Studies have shown that lead can leach from perovskite materials under certain conditions, posing risks to aquatic and terrestrial organisms .
- Degradation Products : The degradation of MAPbI3 can result in the formation of toxic by-products such as lead iodide (PbI2) and methylamine. These degradation products can further interact with biological systems, potentially leading to adverse effects .
Case Studies
- Environmental Impact Studies : Research has indicated that under simulated environmental conditions, MAPbI3 can degrade into lead-containing compounds that are harmful to aquatic life. For instance, a study demonstrated that leaching from perovskite solar cells could result in concentrations of lead that exceed safe limits for freshwater ecosystems .
- Cellular Toxicity Assays : In vitro studies have assessed the cytotoxicity of MAPbI3 and its degradation products on various cell lines. Results indicated that exposure to lead ions released from degraded MAPbI3 can induce oxidative stress and apoptosis in human cell lines .
Comparative Analysis
The following table summarizes key research findings related to the biological activity and toxicity of MAPbI3 compared to other common materials used in solar cells:
Material | Toxicity Level | Degradation Products | Environmental Impact |
---|---|---|---|
CH3NH3PbI3 | High | PbI2, Methylamine | Significant risk to aquatic life |
Silicon | Low | None | Minimal environmental impact |
Cadmium Telluride | Moderate | Cadmium | Moderate risk to soil organisms |
Organic Photovoltaics | Low | Varies | Low risk but variable |
Lead Ion Release Mechanism
The release of lead ions from MAPbI3 occurs through several pathways:
- Photodegradation : Exposure to light can accelerate the breakdown of MAPbI3, leading to increased leaching of lead ions into the environment.
- Hydrolytic Degradation : In humid conditions, the interaction with water can facilitate the conversion of MAPbI3 into soluble lead compounds .
Cellular Mechanisms
Upon exposure to lead ions:
Properties
IUPAC Name |
lead(2+);methylazanium;triiodide | |
---|---|---|
Details | Computed by Lexichem TK 2.7.0 (PubChem release 2021.10.14) | |
Source | PubChem | |
URL | https://pubchem.ncbi.nlm.nih.gov | |
Description | Data deposited in or computed by PubChem | |
InChI |
InChI=1S/CH5N.3HI.Pb/c1-2;;;;/h2H2,1H3;3*1H;/q;;;;+2/p-2 | |
Details | Computed by InChI 1.0.6 (PubChem release 2021.10.14) | |
Source | PubChem | |
URL | https://pubchem.ncbi.nlm.nih.gov | |
Description | Data deposited in or computed by PubChem | |
InChI Key |
OAVDNKDAQKEIHT-UHFFFAOYSA-L | |
Details | Computed by InChI 1.0.6 (PubChem release 2021.10.14) | |
Source | PubChem | |
URL | https://pubchem.ncbi.nlm.nih.gov | |
Description | Data deposited in or computed by PubChem | |
Canonical SMILES |
C[NH3+].[I-].[I-].[I-].[Pb+2] | |
Details | Computed by OEChem 2.3.0 (PubChem release 2021.10.14) | |
Source | PubChem | |
URL | https://pubchem.ncbi.nlm.nih.gov | |
Description | Data deposited in or computed by PubChem | |
Molecular Formula |
CH6I3NPb | |
Details | Computed by PubChem 2.2 (PubChem release 2021.10.14) | |
Source | PubChem | |
URL | https://pubchem.ncbi.nlm.nih.gov | |
Description | Data deposited in or computed by PubChem | |
DSSTOX Substance ID |
DTXSID501338968 | |
Record name | Methanaminium triiodoplumbate(1-) | |
Source | EPA DSSTox | |
URL | https://comptox.epa.gov/dashboard/DTXSID501338968 | |
Description | DSSTox provides a high quality public chemistry resource for supporting improved predictive toxicology. | |
Molecular Weight |
620 g/mol | |
Details | Computed by PubChem 2.2 (PubChem release 2021.10.14) | |
Source | PubChem | |
URL | https://pubchem.ncbi.nlm.nih.gov | |
Description | Data deposited in or computed by PubChem | |
CAS No. |
69507-98-8 | |
Record name | Methanaminium triiodoplumbate(1-) | |
Source | EPA DSSTox | |
URL | https://comptox.epa.gov/dashboard/DTXSID501338968 | |
Description | DSSTox provides a high quality public chemistry resource for supporting improved predictive toxicology. | |
Record name | 69507-98-8 | |
Source | European Chemicals Agency (ECHA) | |
URL | https://echa.europa.eu/information-on-chemicals | |
Description | The European Chemicals Agency (ECHA) is an agency of the European Union which is the driving force among regulatory authorities in implementing the EU's groundbreaking chemicals legislation for the benefit of human health and the environment as well as for innovation and competitiveness. | |
Explanation | Use of the information, documents and data from the ECHA website is subject to the terms and conditions of this Legal Notice, and subject to other binding limitations provided for under applicable law, the information, documents and data made available on the ECHA website may be reproduced, distributed and/or used, totally or in part, for non-commercial purposes provided that ECHA is acknowledged as the source: "Source: European Chemicals Agency, http://echa.europa.eu/". Such acknowledgement must be included in each copy of the material. ECHA permits and encourages organisations and individuals to create links to the ECHA website under the following cumulative conditions: Links can only be made to webpages that provide a link to the Legal Notice page. | |
Disclaimer and Information on In-Vitro Research Products
Please be aware that all articles and product information presented on BenchChem are intended solely for informational purposes. The products available for purchase on BenchChem are specifically designed for in-vitro studies, which are conducted outside of living organisms. In-vitro studies, derived from the Latin term "in glass," involve experiments performed in controlled laboratory settings using cells or tissues. It is important to note that these products are not categorized as medicines or drugs, and they have not received approval from the FDA for the prevention, treatment, or cure of any medical condition, ailment, or disease. We must emphasize that any form of bodily introduction of these products into humans or animals is strictly prohibited by law. It is essential to adhere to these guidelines to ensure compliance with legal and ethical standards in research and experimentation.