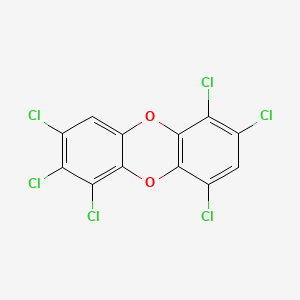
1,2,3,6,7,9-Hexachlorodibenzo-P-dioxin
Overview
Description
1,2,3,6,7,9-Hexachlorodibenzo-P-dioxin is a chlorinated dibenzo-p-dioxin (CDD) isomer. It is a persistent organic pollutant (POP) known for its environmental persistence and potential health impacts.
Preparation Methods
The synthesis of 1,2,3,6,7,9-Hexachlorodibenzo-P-dioxin typically involves the chlorination of dibenzo-p-dioxin under controlled conditions. Industrial production methods often involve high-temperature processes where organic materials containing chlorine are subjected to combustion or chemical reactions. High-resolution gas chromatography and mass spectrometry (HRGC/HRMS) are commonly used to detect and quantify this compound in environmental samples .
Chemical Reactions Analysis
1,2,3,6,7,9-Hexachlorodibenzo-P-dioxin undergoes various chemical reactions, including:
Oxidation: This reaction can lead to the formation of more chlorinated dioxins or other oxidized products.
Reduction: Under specific conditions, the compound can be reduced to less chlorinated forms.
Substitution: Chlorine atoms in the molecule can be substituted with other atoms or groups under appropriate conditions. Common reagents used in these reactions include strong oxidizing agents, reducing agents, and catalysts. .
Scientific Research Applications
1,2,3,6,7,9-Hexachlorodibenzo-P-dioxin is primarily studied for its environmental and health impacts. It is used in research to understand the mechanisms of toxicity and the effects of long-term exposure to PCDDs. Studies often focus on its role as a persistent organic pollutant, its bioaccumulation in the food chain, and its potential to cause cancer and other health issues .
Mechanism of Action
The compound exerts its effects by binding to the aryl hydrocarbon receptor (AhR), a ligand-activated transcription factor. This binding leads to the activation of various genes involved in the metabolism of xenobiotics, including phase I and II detoxification enzymes such as CYP1A1. The activation of these pathways can result in toxic effects, including disruption of cell cycle regulation and induction of oxidative stress .
Comparison with Similar Compounds
1,2,3,6,7,9-Hexachlorodibenzo-P-dioxin is similar to other PCDDs, such as:
- 1,2,3,7,8,9-Hexachlorodibenzo-P-dioxin
- 1,2,3,6,7,8-Hexachlorodibenzo-P-dioxin
- 2,3,7,8-Tetrachlorodibenzo-P-dioxin (TCDD) These compounds share similar structures and toxicological properties but differ in the number and positions of chlorine atoms. This compound is unique due to its specific chlorine substitution pattern, which influences its chemical reactivity and biological effects .
Biological Activity
1,2,3,6,7,9-Hexachlorodibenzo-P-dioxin (HxCDD) is a member of the dioxin family, known for its environmental persistence and potential health effects. This article explores its biological activity, including toxicity, carcinogenicity, metabolism, and environmental impact.
Chemical Structure and Properties
HxCDD is characterized by its six chlorine atoms attached to a dibenzo-p-dioxin structure. Its molecular formula is C12H4Cl6O2, and it has a high lipophilicity, which contributes to its bioaccumulation in living organisms.
Toxicological Effects
Acute and Chronic Toxicity:
HxCDD exhibits significant toxicity in various animal models. Studies have shown that exposure to HxCDD leads to:
- Weight Loss: A dose-dependent decrease in body weight was observed in rodent models following exposure to HxCDD. For instance, male and female rats treated with doses of 80 µg/kg exhibited substantial weight loss compared to controls .
- Liver Damage: Histopathological examinations revealed degenerative liver changes and necrosis in rats and mice exposed to HxCDD. Notably, there was a significant increase in hepatocellular carcinomas in male rats at higher doses .
Table 1: Summary of Toxicological Findings
Study Type | Organism | Dose (µg/kg) | Observed Effect |
---|---|---|---|
Carcinogenicity Study | Osborne-Mendel Rats | 1.25 - 5.0 | Increased liver tumors |
Dermal Study | Swiss-Webster Mice | 0.005 - 0.01 | No significant carcinogenic response |
Weight Gain Study | Sprague-Dawley Rats | 0.05 - 80 | Dose-dependent weight loss |
Carcinogenic Potential
The National Toxicology Program (NTP) conducted studies indicating that HxCDD has a potential carcinogenic effect. In male rats, there was a dose-related increase in hepatocellular carcinomas and neoplastic nodules following prolonged exposure . The study concluded that while evidence for carcinogenicity was inconclusive for male rats, female rats showed a clear increase in liver tumors at medium and high doses.
Metabolism and Biotransformation
Research indicates that HxCDD undergoes biotransformation primarily through microbial action. For example:
- Bacterial Catabolism: A study demonstrated that certain bacteria could degrade HxCDD into less chlorinated metabolites such as tetrachlorocatechol and tetrachlorophenol. This process is crucial for understanding how HxCDD can be biologically transformed in contaminated environments .
- Influence of Substitution Patterns: The metabolic pathways of HxCDD are influenced by the specific chlorine substitution pattern on the dioxin molecule. This affects both its toxicity and biodegradability .
Environmental Impact
HxCDD is persistent in the environment due to its stability and resistance to degradation. It is often found in:
- Soil and Sediments: Contamination from industrial processes and the use of certain pesticides has led to detectable levels of HxCDD in various ecosystems.
- Bioaccumulation: Due to its lipophilic nature, HxCDD accumulates in the fatty tissues of organisms, leading to biomagnification through food webs.
Table 2: Environmental Persistence
Environmental Matrix | Concentration (pg/g) | Source of Contamination |
---|---|---|
Soil | 84.1 | Pesticides |
Sediment | Varies | Industrial discharge |
Aquatic Organisms | High levels | Bioaccumulation from contaminated water |
Case Studies
Several case studies have documented the effects of HxCDD exposure:
- Industrial Exposure: Workers at chemical manufacturing plants exhibited elevated levels of dioxins in their blood, correlating with increased incidences of liver disease and cancer.
- Environmental Remediation Projects: Sites contaminated with HxCDD have been subject to bioremediation efforts using specific bacterial strains capable of degrading dioxins effectively.
Properties
IUPAC Name |
1,2,3,6,7,9-hexachlorodibenzo-p-dioxin | |
---|---|---|
Details | Computed by Lexichem TK 2.7.0 (PubChem release 2021.05.07) | |
Source | PubChem | |
URL | https://pubchem.ncbi.nlm.nih.gov | |
Description | Data deposited in or computed by PubChem | |
InChI |
InChI=1S/C12H2Cl6O2/c13-3-1-5(15)10-12(8(3)17)19-6-2-4(14)7(16)9(18)11(6)20-10/h1-2H | |
Details | Computed by InChI 1.0.6 (PubChem release 2021.05.07) | |
Source | PubChem | |
URL | https://pubchem.ncbi.nlm.nih.gov | |
Description | Data deposited in or computed by PubChem | |
InChI Key |
BQOHWGKNRKCEFT-UHFFFAOYSA-N | |
Details | Computed by InChI 1.0.6 (PubChem release 2021.05.07) | |
Source | PubChem | |
URL | https://pubchem.ncbi.nlm.nih.gov | |
Description | Data deposited in or computed by PubChem | |
Canonical SMILES |
C1=C2C(=C(C(=C1Cl)Cl)Cl)OC3=C(O2)C(=C(C=C3Cl)Cl)Cl | |
Details | Computed by OEChem 2.3.0 (PubChem release 2021.05.07) | |
Source | PubChem | |
URL | https://pubchem.ncbi.nlm.nih.gov | |
Description | Data deposited in or computed by PubChem | |
Molecular Formula |
C12H2Cl6O2 | |
Details | Computed by PubChem 2.1 (PubChem release 2021.05.07) | |
Source | PubChem | |
URL | https://pubchem.ncbi.nlm.nih.gov | |
Description | Data deposited in or computed by PubChem | |
DSSTOX Substance ID |
DTXSID3074074 | |
Record name | 1,2,3,6,7,9-Hexachlorodibenzo-p-dioxin | |
Source | EPA DSSTox | |
URL | https://comptox.epa.gov/dashboard/DTXSID3074074 | |
Description | DSSTox provides a high quality public chemistry resource for supporting improved predictive toxicology. | |
Molecular Weight |
390.9 g/mol | |
Details | Computed by PubChem 2.1 (PubChem release 2021.05.07) | |
Source | PubChem | |
URL | https://pubchem.ncbi.nlm.nih.gov | |
Description | Data deposited in or computed by PubChem | |
CAS No. |
64461-98-9, 58802-51-0 | |
Record name | 1,2,3,6,7,9-Hexachlorodibenzo-p-dioxin | |
Source | CAS Common Chemistry | |
URL | https://commonchemistry.cas.org/detail?cas_rn=64461-98-9 | |
Description | CAS Common Chemistry is an open community resource for accessing chemical information. Nearly 500,000 chemical substances from CAS REGISTRY cover areas of community interest, including common and frequently regulated chemicals, and those relevant to high school and undergraduate chemistry classes. This chemical information, curated by our expert scientists, is provided in alignment with our mission as a division of the American Chemical Society. | |
Explanation | The data from CAS Common Chemistry is provided under a CC-BY-NC 4.0 license, unless otherwise stated. | |
Record name | Dibenzo-p-dioxin, 1,2,3,4,5,7-hexachloro- | |
Source | ChemIDplus | |
URL | https://pubchem.ncbi.nlm.nih.gov/substance/?source=chemidplus&sourceid=0058802510 | |
Description | ChemIDplus is a free, web search system that provides access to the structure and nomenclature authority files used for the identification of chemical substances cited in National Library of Medicine (NLM) databases, including the TOXNET system. | |
Record name | 1,2,3,6,7,9-Hexachlorodibenzo-p-dioxin | |
Source | ChemIDplus | |
URL | https://pubchem.ncbi.nlm.nih.gov/substance/?source=chemidplus&sourceid=0064461989 | |
Description | ChemIDplus is a free, web search system that provides access to the structure and nomenclature authority files used for the identification of chemical substances cited in National Library of Medicine (NLM) databases, including the TOXNET system. | |
Record name | 1,2,3,6,7,9-Hexachlorodibenzo-p-dioxin | |
Source | EPA DSSTox | |
URL | https://comptox.epa.gov/dashboard/DTXSID3074074 | |
Description | DSSTox provides a high quality public chemistry resource for supporting improved predictive toxicology. | |
Record name | 1,2,3,6,7,9-HEXACHLORODIBENZO-P-DIOXIN | |
Source | FDA Global Substance Registration System (GSRS) | |
URL | https://gsrs.ncats.nih.gov/ginas/app/beta/substances/M2S20KFX8M | |
Description | The FDA Global Substance Registration System (GSRS) enables the efficient and accurate exchange of information on what substances are in regulated products. Instead of relying on names, which vary across regulatory domains, countries, and regions, the GSRS knowledge base makes it possible for substances to be defined by standardized, scientific descriptions. | |
Explanation | Unless otherwise noted, the contents of the FDA website (www.fda.gov), both text and graphics, are not copyrighted. They are in the public domain and may be republished, reprinted and otherwise used freely by anyone without the need to obtain permission from FDA. Credit to the U.S. Food and Drug Administration as the source is appreciated but not required. | |
Retrosynthesis Analysis
AI-Powered Synthesis Planning: Our tool employs the Template_relevance Pistachio, Template_relevance Bkms_metabolic, Template_relevance Pistachio_ringbreaker, Template_relevance Reaxys, Template_relevance Reaxys_biocatalysis model, leveraging a vast database of chemical reactions to predict feasible synthetic routes.
One-Step Synthesis Focus: Specifically designed for one-step synthesis, it provides concise and direct routes for your target compounds, streamlining the synthesis process.
Accurate Predictions: Utilizing the extensive PISTACHIO, BKMS_METABOLIC, PISTACHIO_RINGBREAKER, REAXYS, REAXYS_BIOCATALYSIS database, our tool offers high-accuracy predictions, reflecting the latest in chemical research and data.
Strategy Settings
Precursor scoring | Relevance Heuristic |
---|---|
Min. plausibility | 0.01 |
Model | Template_relevance |
Template Set | Pistachio/Bkms_metabolic/Pistachio_ringbreaker/Reaxys/Reaxys_biocatalysis |
Top-N result to add to graph | 6 |
Feasible Synthetic Routes
Disclaimer and Information on In-Vitro Research Products
Please be aware that all articles and product information presented on BenchChem are intended solely for informational purposes. The products available for purchase on BenchChem are specifically designed for in-vitro studies, which are conducted outside of living organisms. In-vitro studies, derived from the Latin term "in glass," involve experiments performed in controlled laboratory settings using cells or tissues. It is important to note that these products are not categorized as medicines or drugs, and they have not received approval from the FDA for the prevention, treatment, or cure of any medical condition, ailment, or disease. We must emphasize that any form of bodily introduction of these products into humans or animals is strictly prohibited by law. It is essential to adhere to these guidelines to ensure compliance with legal and ethical standards in research and experimentation.