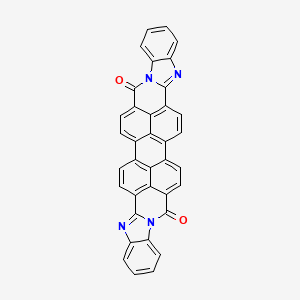
Perylenebisimide with extended PI system
Overview
Description
Perylenebisimide with extended PI system is a compound known for its unique electronic properties and extensive applications in various fields. . These properties make it a valuable material in organic electronics, dyes, pigments, and sensors.
Mechanism of Action
Target of Action
The primary target of Perylenebisimide (PBI) with an extended π system is organic molecules . The compound is used for label-free sensing of these molecules . The extended π system of the PBI backbone strongly binds the analytes .
Mode of Action
PBI interacts with its targets (organic molecules) by changing its electronic properties . This change is investigated in five symmetric and asymmetric PBI derivatives, which share a common backbone but are functionalised with various bay-area substituents . The change in conductance in response to the binding of three analytes, namely TNT, BEDT-TTF, and TCNE, provides a unique fingerprint for the discriminating sensing of each analyte .
Biochemical Pathways
The biochemical pathways affected by PBI are primarily related to the electronic properties of the organic molecules it targets . The change in these properties leads to a unique electrical response to analyte binding .
Result of Action
The result of PBI’s action is a unique electrical response to analyte binding . This response is a direct consequence of the extended π system of the PBI backbone, which strongly binds the analytes, combined with the different charge distribution of the five PBI derivatives .
Biochemical Analysis
Biochemical Properties
Perylenebisimide with an extended PI system plays a crucial role in biochemical reactions, particularly in the context of sensing and molecular recognition. This compound interacts with a variety of enzymes, proteins, and other biomolecules through non-covalent interactions such as π-π stacking and hydrogen bonding. For instance, it has been shown to bind strongly to analytes like TNT, BEDT-TTF, and TCNE, providing a unique electrical response that can be used for label-free sensing . These interactions are facilitated by the extended PI system, which allows for a larger surface area for binding and enhances the compound’s sensitivity and selectivity.
Cellular Effects
The effects of perylenebisimide with an extended PI system on cellular processes are profound. This compound influences cell function by modulating cell signaling pathways, gene expression, and cellular metabolism. It has been observed that perylenebisimide derivatives can affect the electrical conductance of cells, which is crucial for understanding their role in cellular communication and signal transduction . Additionally, the compound’s ability to interact with various biomolecules can lead to changes in gene expression, further impacting cellular metabolism and function.
Molecular Mechanism
At the molecular level, perylenebisimide with an extended PI system exerts its effects through specific binding interactions with biomolecules. The extended PI system allows for strong π-π stacking interactions with aromatic amino acids in proteins, leading to enzyme inhibition or activation. This compound can also modulate gene expression by binding to DNA or RNA, affecting transcription and translation processes . The unique electronic properties of perylenebisimide enable it to act as an efficient electron transporter, further influencing biochemical pathways.
Temporal Effects in Laboratory Settings
In laboratory settings, the effects of perylenebisimide with an extended PI system can change over time. The compound exhibits high stability and resistance to degradation, maintaining its activity over extended periods . Long-term studies have shown that perylenebisimide can have sustained effects on cellular function, including prolonged modulation of cell signaling pathways and gene expression. The compound’s stability can be influenced by environmental factors such as temperature and pH, which should be carefully controlled during experiments.
Dosage Effects in Animal Models
The effects of perylenebisimide with an extended PI system vary with different dosages in animal models. At low doses, the compound can enhance cellular function and promote beneficial biochemical reactions. At high doses, it may exhibit toxic or adverse effects, such as disrupting cellular homeostasis and causing oxidative stress . Threshold effects have been observed, indicating that there is an optimal dosage range for achieving the desired biochemical outcomes without causing harm.
Metabolic Pathways
Perylenebisimide with an extended PI system is involved in several metabolic pathways, interacting with enzymes and cofactors that regulate cellular metabolism. The compound can influence metabolic flux by modulating the activity of key enzymes, leading to changes in metabolite levels . For example, it has been shown to affect the electron transport chain in mitochondria, impacting ATP production and overall cellular energy metabolism.
Transport and Distribution
Within cells and tissues, perylenebisimide with an extended PI system is transported and distributed through interactions with specific transporters and binding proteins. These interactions facilitate the compound’s localization to target sites, where it can exert its biochemical effects . The extended PI system enhances the compound’s ability to penetrate cellular membranes and accumulate in specific compartments, such as the nucleus or mitochondria.
Subcellular Localization
The subcellular localization of perylenebisimide with an extended PI system is critical for its activity and function. The compound is often directed to specific compartments or organelles through targeting signals or post-translational modifications . For instance, it may localize to the nucleus to modulate gene expression or to the mitochondria to influence cellular metabolism. The extended PI system plays a key role in determining the compound’s localization and its subsequent biochemical effects.
Preparation Methods
The synthesis of perylenebisimide with extended PI system typically involves the condensation of perylene-3,4,9,10-tetracarboxylic dianhydride with primary amines . The reaction is usually carried out in a solvent such as N,N-dimethylformamide (DMF) or N-methyl-2-pyrrolidone (NMP) at elevated temperatures. Industrial production methods often involve similar synthetic routes but are optimized for large-scale production, ensuring high yield and purity of the final product .
Chemical Reactions Analysis
Perylenebisimide with extended PI system undergoes various chemical reactions, including:
Oxidation: This compound can be oxidized to form perylene bisimide derivatives with different electronic properties.
Reduction: Reduction reactions can lead to the formation of reduced perylene bisimide species, which have distinct optical and electronic characteristics.
Common reagents used in these reactions include oxidizing agents like potassium permanganate, reducing agents such as sodium borohydride, and various nucleophiles for substitution reactions. The major products formed from these reactions are typically derivatives of perylene bisimide with modified electronic and optical properties .
Scientific Research Applications
Perylenebisimide with extended PI system has a wide range of scientific research applications:
Chemistry: It is used as a building block for the synthesis of complex organic molecules and materials.
Biology: The compound’s fluorescence properties make it useful in biological imaging and as a fluorescent probe.
Medicine: Perylenebisimide derivatives are explored for their potential in photodynamic therapy and as drug delivery agents.
Comparison with Similar Compounds
Perylenebisimide with extended PI system is unique due to its large π-system and high electron affinity. Similar compounds include:
Naphthalene diimides: These compounds have smaller π-systems and lower electron affinities compared to perylenebisimide.
Anthracene diimides: Similar to naphthalene diimides, these compounds also have smaller π-systems and are less stable than perylenebisimide.
Perylene tetracarboxylic dianhydride: This compound is a precursor to perylenebisimide and shares some of its properties but lacks the extended π-system.
This compound stands out due to its superior electronic properties, making it a preferred choice for applications in organic electronics and sensing technologies .
Properties
IUPAC Name |
7,14,25,32-tetrazaundecacyclo[21.13.2.22,5.03,19.04,16.06,14.08,13.020,37.024,32.026,31.034,38]tetraconta-1(36),2,4,6,8,10,12,16,18,20(37),21,23(38),24,26,28,30,34,39-octadecaene-15,33-dione | |
---|---|---|
Details | Computed by Lexichem TK 2.7.0 (PubChem release 2021.05.07) | |
Source | PubChem | |
URL | https://pubchem.ncbi.nlm.nih.gov | |
Description | Data deposited in or computed by PubChem | |
InChI |
InChI=1S/C36H16N4O2/c41-35-23-15-11-19-18-10-14-22-32-24(36(42)40-28-8-4-2-6-26(28)38-34(22)40)16-12-20(30(18)32)17-9-13-21(31(23)29(17)19)33-37-25-5-1-3-7-27(25)39(33)35/h1-16H | |
Details | Computed by InChI 1.0.6 (PubChem release 2021.05.07) | |
Source | PubChem | |
URL | https://pubchem.ncbi.nlm.nih.gov | |
Description | Data deposited in or computed by PubChem | |
InChI Key |
CNXXSKARMYGQBG-UHFFFAOYSA-N | |
Details | Computed by InChI 1.0.6 (PubChem release 2021.05.07) | |
Source | PubChem | |
URL | https://pubchem.ncbi.nlm.nih.gov | |
Description | Data deposited in or computed by PubChem | |
Canonical SMILES |
C1=CC=C2C(=C1)N=C3N2C(=O)C4=CC=C5C6=C7C(=CC=C8C7=C(C=C6)C9=NC1=CC=CC=C1N9C8=O)C1=C5C4=C3C=C1 | |
Details | Computed by OEChem 2.3.0 (PubChem release 2021.05.07) | |
Source | PubChem | |
URL | https://pubchem.ncbi.nlm.nih.gov | |
Description | Data deposited in or computed by PubChem | |
Molecular Formula |
C36H16N4O2 | |
Details | Computed by PubChem 2.1 (PubChem release 2021.05.07) | |
Source | PubChem | |
URL | https://pubchem.ncbi.nlm.nih.gov | |
Description | Data deposited in or computed by PubChem | |
DSSTOX Substance ID |
DTXSID801146683 | |
Record name | N,N′-Diphenylglyoxaline-3,4,9,10-perylenetetracarboxylic acid diacidamide | |
Source | EPA DSSTox | |
URL | https://comptox.epa.gov/dashboard/DTXSID801146683 | |
Description | DSSTox provides a high quality public chemistry resource for supporting improved predictive toxicology. | |
Molecular Weight |
536.5 g/mol | |
Details | Computed by PubChem 2.1 (PubChem release 2021.05.07) | |
Source | PubChem | |
URL | https://pubchem.ncbi.nlm.nih.gov | |
Description | Data deposited in or computed by PubChem | |
CAS No. |
55034-79-2 | |
Record name | N,N′-Diphenylglyoxaline-3,4,9,10-perylenetetracarboxylic acid diacidamide | |
Source | CAS Common Chemistry | |
URL | https://commonchemistry.cas.org/detail?cas_rn=55034-79-2 | |
Description | CAS Common Chemistry is an open community resource for accessing chemical information. Nearly 500,000 chemical substances from CAS REGISTRY cover areas of community interest, including common and frequently regulated chemicals, and those relevant to high school and undergraduate chemistry classes. This chemical information, curated by our expert scientists, is provided in alignment with our mission as a division of the American Chemical Society. | |
Explanation | The data from CAS Common Chemistry is provided under a CC-BY-NC 4.0 license, unless otherwise stated. | |
Record name | N,N′-Diphenylglyoxaline-3,4,9,10-perylenetetracarboxylic acid diacidamide | |
Source | EPA DSSTox | |
URL | https://comptox.epa.gov/dashboard/DTXSID801146683 | |
Description | DSSTox provides a high quality public chemistry resource for supporting improved predictive toxicology. | |
Retrosynthesis Analysis
AI-Powered Synthesis Planning: Our tool employs the Template_relevance Pistachio, Template_relevance Bkms_metabolic, Template_relevance Pistachio_ringbreaker, Template_relevance Reaxys, Template_relevance Reaxys_biocatalysis model, leveraging a vast database of chemical reactions to predict feasible synthetic routes.
One-Step Synthesis Focus: Specifically designed for one-step synthesis, it provides concise and direct routes for your target compounds, streamlining the synthesis process.
Accurate Predictions: Utilizing the extensive PISTACHIO, BKMS_METABOLIC, PISTACHIO_RINGBREAKER, REAXYS, REAXYS_BIOCATALYSIS database, our tool offers high-accuracy predictions, reflecting the latest in chemical research and data.
Strategy Settings
Precursor scoring | Relevance Heuristic |
---|---|
Min. plausibility | 0.01 |
Model | Template_relevance |
Template Set | Pistachio/Bkms_metabolic/Pistachio_ringbreaker/Reaxys/Reaxys_biocatalysis |
Top-N result to add to graph | 6 |
Feasible Synthetic Routes
Disclaimer and Information on In-Vitro Research Products
Please be aware that all articles and product information presented on BenchChem are intended solely for informational purposes. The products available for purchase on BenchChem are specifically designed for in-vitro studies, which are conducted outside of living organisms. In-vitro studies, derived from the Latin term "in glass," involve experiments performed in controlled laboratory settings using cells or tissues. It is important to note that these products are not categorized as medicines or drugs, and they have not received approval from the FDA for the prevention, treatment, or cure of any medical condition, ailment, or disease. We must emphasize that any form of bodily introduction of these products into humans or animals is strictly prohibited by law. It is essential to adhere to these guidelines to ensure compliance with legal and ethical standards in research and experimentation.