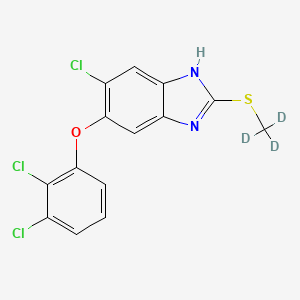
Triclabendazole-D3
Overview
Description
Triclabendazole-D3 (C₁₄H₆D₃Cl₃N₂OS) is a deuterium-labeled analog of triclabendazole, a benzimidazole anthelmintic used primarily in veterinary medicine to treat parasitic infections. The deuterated form replaces three hydrogen atoms with deuterium at the methylsulfinyl group, enhancing its utility as an internal standard in mass spectrometry-based analytical methods . Key properties include:
Property | Value |
---|---|
Molecular Weight | 362.68 g/mol |
CAS Number | 1353867-93-2 |
Density | 1.6 ± 0.1 g/cm³ |
Boiling Point | 495.9 ± 55.0 °C |
LogP (Partition Coefficient) | 5.97 |
Storage Conditions | 2–8°C in a closed, light-protected container |
This compound is critical for quantifying parent drug residues in biological matrices, ensuring compliance with food safety regulations (e.g., EU Maximum Residue Limits) . Its deuterated structure minimizes isotopic interference, improving analytical precision in liquid chromatography-tandem mass spectrometry (LC-MS/MS) .
Preparation Methods
The synthesis of triclabendazole involves several steps, starting from 3,4-dichloroaniline. The process includes acylation, nitration, hydrolysis, etherification, reduction, and cyclization . The final step involves methylation using dimethyl sulfate . This method avoids the use of hazardous materials like sodium and high-pressure reactions, making it more environmentally friendly and suitable for industrial production .
Chemical Reactions Analysis
Triclabendazole undergoes several types of chemical reactions:
Oxidation: Triclabendazole is metabolized into sulfone and sulfoxide metabolites through oxidation.
Reduction: The reduction of nitro groups during synthesis is a crucial step.
Substitution: Etherification involves the substitution of hydrogen atoms with alkoxy groups.
Common reagents used in these reactions include dimethyl sulfate for methylation and various acids and bases for hydrolysis and etherification . The major products formed are the sulfone and sulfoxide metabolites, which are active against both immature and mature liver flukes .
Scientific Research Applications
Pharmacokinetics and Metabolism
Triclabendazole-D3 is utilized in pharmacokinetic studies to understand its absorption, distribution, metabolism, and excretion (ADME) properties. The deuterated form allows researchers to trace the compound more effectively in biological systems due to the distinct mass difference between deuterium and hydrogen.
- Metabolite Analysis : Studies have shown that triclabendazole undergoes extensive metabolism, primarily converting into its sulfoxide and sulfone metabolites. The pharmacokinetics of these metabolites are crucial for understanding the drug's efficacy and safety profile. For instance, the sulfoxide metabolite is believed to be more potent than the parent compound in terms of its effect on parasite motility .
- Analytical Techniques : Liquid chromatography-tandem mass spectrometry (LC-MS/MS) has been developed for the rapid determination of triclabendazole and its metabolites in biological matrices such as blood and tissue samples. This method provides high sensitivity and specificity, essential for pharmacokinetic studies .
Clinical Applications
This compound has been extensively studied in clinical settings for its effectiveness against various parasitic infections.
- Fascioliasis Treatment : Clinical trials have demonstrated that triclabendazole is effective against all stages of Fasciola infections. A review of multiple studies indicates that a dosage regimen of 10 mg/kg or 20 mg/kg leads to high cure rates (ranging from 70% to 100%) in patients with fascioliasis .
- Comparative Studies : In a randomized trial comparing triclabendazole with artesunate for acute fascioliasis, results showed that while artesunate had a higher immediate resolution of symptoms, triclabendazole yielded a better long-term response at three months post-treatment .
Analytical Method Development
The development of analytical methods for detecting this compound is crucial for ensuring food safety and regulatory compliance.
- Residue Detection : Research has focused on detecting residues of triclabendazole in animal tissues (e.g., bovine and goat muscle). A validated LC-MS/MS method demonstrated recoveries between 96.1% and 105.6%, indicating its reliability for monitoring drug residues in food products intended for human consumption .
- Quality Control : this compound is also employed in quality control applications within pharmaceutical manufacturing to ensure that products meet safety standards before reaching consumers .
Case Studies
Several case studies illustrate the practical applications of this compound in veterinary medicine and human health:
- Veterinary Use : In sheep, the combined use of ivermectin and triclabendazole has been studied to assess their pharmacological interactions. This research helps optimize treatment protocols for parasitic infections in livestock .
- Human Health : A comprehensive review of clinical data over nearly three decades highlights the consistent efficacy of triclabendazole in treating human fascioliasis across various demographics, including adults and children . However, there remains a lack of data on its use in vulnerable populations such as pregnant women or those with renal impairment.
Mechanism of Action
Triclabendazole and its metabolites are absorbed by the tegument of liver flukes, leading to a decrease in the resting membrane potential, inhibition of motility, and disruption of the surface ultrastructure . This includes the inhibition of spermatogenesis and vitelline cells . The drug also induces pyroptosis in cancer cells by activating caspase-3, leading to the cleavage of gasdermin E .
Comparison with Similar Compounds
Comparison with Similar Compounds
Structural and Functional Analogues
Deuterated Benzimidazoles
Triclabendazole-D3 belongs to a class of deuterated benzimidazoles used as internal standards. Below is a comparative analysis:
Compound | Molecular Formula | CAS No. | Key Application |
---|---|---|---|
This compound | C₁₄H₆D₃Cl₃N₂OS | 1353867-93-2 | Quantification of triclabendazole residues |
Albendazole-D3 | C₁₂H₁₂D₃N₃O₂S | N/A | Albendazole residue analysis in livestock |
Mebendazole-D3 | C₁₆H₁₁D₃N₃O₃ | N/A | Detection of mebendazole in food matrices |
Flubendazole-D3 | C₁₆H₁₀D₃FN₃O₃ | N/A | Monitoring flubendazole metabolism |
Key Findings :
- All deuterated analogs share a benzimidazole backbone but differ in substituents (e.g., Cl, F, or methyl groups), affecting their specificity in targeting parent drugs .
- This compound exhibits superior stability in organic solvents (e.g., methanol, DMSO) compared to non-deuterated forms, reducing matrix effects in LC-MS/MS .
Non-Deuterated Structural Analogues
Compound | Structure | Role |
---|---|---|
Triclabendazole Sulfone | C₁₄H₉Cl₃N₂O₃S | Oxidative metabolite for residue monitoring |
Triclabendazole Sulfoxide | C₁₄H₉Cl₃N₂O₂S | Primary pharmacologically active metabolite |
4-Chloro-5-(2,3-dichlorophenoxy)-2-nitroaniline | C₁₂H₇Cl₃N₂O₃ | Degradation product (USP Reference Standard) |
Key Findings :
- Triclabendazole sulfoxide-D3 (CAS: 100648-13-3) is another deuterated metabolite used to track drug biotransformation .
- Non-deuterated analogues like triclabendazole sulfone are essential for validating analytical methods but lack isotopic distinction, limiting their use as internal standards .
Analytical Performance Comparison
Sensitivity and Selectivity in LC-MS/MS
Compound | Limit of Detection (LOD) | Recovery Rate |
---|---|---|
This compound | 2 pg/mL | 98–102% |
Albendazole-D3 | 4 pg/mL | 95–98% |
Mebendazole-D3 | 3 pg/mL | 97–101% |
Research Insights :
- This compound demonstrates lower LODs than albendazole-D3 due to reduced ion suppression in complex matrices .
- Recovery rates >95% confirm minimal deuterium exchange during extraction, ensuring reliable quantification .
Regulatory and Commercial Landscape
- Suppliers : Witega Laboratories and Quechem Ltd. are primary suppliers of deuterated benzimidazoles, with this compound priced at ~$500/50 mg .
- Regulatory Compliance : Complies with REACH, OECD, and GHS guidelines for stable isotope-labeled compounds .
Research and Development Implications
Biological Activity
Triclabendazole-D3, a deuterated form of triclabendazole, is primarily recognized for its significant biological activity against helminths, particularly liver flukes such as Fasciola hepatica. This article explores its mechanism of action, pharmacokinetics, efficacy in clinical settings, and case studies highlighting treatment outcomes.
This compound is a benzimidazole derivative characterized by the incorporation of three deuterium atoms. This modification enhances its stability and aids in analytical studies. The compound operates by binding to the colchicine site on β-tubulin, disrupting microtubule dynamics crucial for cell division and function. This disruption leads to reduced egg production and viability in infected hosts, making it effective against various parasitic infections.
Pharmacokinetics
The pharmacokinetics of this compound involve several metabolic transformations. Upon administration, it is metabolized into triclabendazole sulfoxide and subsequently into triclabendazole sulfone. The sulfone metabolite is particularly noted for its prolonged action against parasites.
Table 1: Pharmacokinetic Properties of this compound
Parameter | Value |
---|---|
Half-life | Variable (dependent on species) |
Bioavailability | Enhanced with food intake |
Metabolites | Triclabendazole sulfoxide, Triclabendazole sulfone |
Efficacy Against Liver Flukes
Clinical studies have demonstrated the efficacy of this compound in treating infections caused by Fasciola hepatica. A retrospective cohort study involving children with chronic fascioliasis in Peru showed that 55% achieved parasitological cure after the first treatment with a single dose of 10 mg/kg. However, cure rates declined with repeated treatments, indicating potential resistance development .
Table 2: Efficacy Rates by Treatment Round
Treatment Round | Cure Rate (%) | Number of Patients |
---|---|---|
First | 55 | 146 |
Second | 38 | - |
Third | 30 | - |
Fourth | 23 | - |
Case Studies
Several case studies illustrate the complexities of treating Fasciola hepatica infections with this compound:
- Case Study 1 : A 51-year-old female experienced significant weight loss and abdominal pain. Initial treatment with a single dose of triclabendazole led to symptom improvement; however, symptoms recurred after eight months despite multiple treatment courses .
- Case Study 2 : A 36-year-old female presented with jaundice and severe joint pain. After failing multiple courses of triclabendazole, she eventually achieved symptom relief with a combination therapy including nitazoxanide .
- Case Study 3 : In a cohort study of children treated for fascioliasis, those with higher baseline egg counts had lower cure rates, emphasizing the need for careful monitoring and potential alternative therapies for resistant cases .
Q & A
Q. Basic: How can researchers ensure isotopic purity and stability of Triclabendazole-D3 during synthesis?
Methodological Answer:
To validate isotopic purity, employ liquid chromatography-tandem mass spectrometry (LC-MS/MS) with deuterium-specific fragmentation patterns and compare against non-deuterated standards . Stability testing under varying temperatures (4°C, 25°C) and pH conditions (e.g., simulated gastric fluid) should be conducted over 30 days, with periodic sampling analyzed via nuclear magnetic resonance (NMR) to detect proton-deuterium exchange . Use sealed, inert containers to minimize environmental degradation. Document synthesis protocols in triplicate to ensure reproducibility .
Q. Basic: What analytical techniques are optimal for quantifying this compound in biological matrices?
Methodological Answer:
Use high-resolution mass spectrometry (HRMS) coupled with solid-phase extraction (SPE) to isolate the compound from plasma or tissue homogenates. Calibrate with deuterium-enriched internal standards (e.g., Triclabendazole-D6) to correct for matrix effects. Validate sensitivity (limit of quantification ≤1 ng/mL), accuracy (85–115% recovery), and precision (CV <15%) per ICH Q2(R1) guidelines . For tissue distribution studies, combine with autoradiography using radiolabeled analogs .
Q. Advanced: How should researchers address contradictory pharmacokinetic data between in vitro and in vivo models for this compound?
Methodological Answer:
First, conduct a root-cause analysis :
- Compare dissolution profiles (e.g., biorelevant media vs. in vivo fluids) using USP apparatus IV .
- Evaluate species-specific metabolic pathways via hepatic microsomal assays (human vs. rodent CYP450 isoforms) .
- Apply compartmental pharmacokinetic modeling (e.g., NONMEM) to identify absorption barriers or nonlinear clearance .
If discrepancies persist, redesign studies using physiologically based pharmacokinetic (PBPK) models to simulate interspecies differences .
Q. Advanced: What experimental designs minimize bias in assessing this compound resistance in Fasciola hepatica?
Methodological Answer:
Adopt a blinded, randomized controlled trial (RCT) design:
- Stratify parasites by geographic origin and pretreatment susceptibility .
- Expose isolates to incremental this compound concentrations (0.1–10 µg/mL) in triplicate, using lethality assays (e.g., motility scoring, ATP quantification) .
- Validate resistance markers (e.g., β-tubulin mutations) via whole-genome sequencing and correlate with phenotypic data .
- Apply Cochran-Mantel-Haenszel tests to control for confounding variables like egg viability .
Q. Advanced: How can researchers resolve inconsistencies in metabolic pathway identification for this compound across species?
Methodological Answer:
Implement triangulation using three methodologies:
In vitro metabolism : Incubate with liver microsomes from human, rat, and sheep, analyzing metabolites via LC-HRMS .
In silico prediction : Use software like Meteor Nexus to simulate phase I/II transformations .
In vivo corroboration : Administer deuterated compound to animal models, collecting bile/urine for NMR-based structural elucidation .
Discrepancies require enzyme kinetic studies (e.g., Km, Vmax) to identify species-specific catalytic efficiencies .
Q. Basic: What protocols ensure ethical and reproducible dosing in this compound animal studies?
Methodological Answer:
- Follow ARRIVE 2.0 guidelines for dose calculation (mg/kg based on body surface area) and randomization .
- Use pharmacokinetic-guided dosing : Adjust intervals based on trough plasma concentrations measured via LC-MS/MS .
- Document adverse events (e.g., hepatotoxicity) using histopathology and serum biomarkers (ALT, AST) .
- Share raw data in FAIR-aligned repositories (e.g., Zenodo) to enable meta-analyses .
Q. Advanced: How to optimize study designs for cross-species extrapolation of this compound efficacy?
Methodological Answer:
Employ allometric scaling adjusted for metabolic rate differences (e.g., human vs. cattle). Validate using:
- Interspecies correlation plots for AUC and Cmax .
- Monte Carlo simulations to predict clinical outcomes from preclinical data .
For parasiticidal activity, integrate time-kill curves and subpopulation modeling to account for heteroresistance .
Q. Advanced: What statistical methods address variability in this compound’s bioavailability studies?
Methodological Answer:
- Apply mixed-effects models (e.g., SAS PROC MIXED) to partition variability into inter-subject, intra-subject, and formulation effects .
- Use bootstrap resampling to estimate confidence intervals for bioavailability ratios .
- For food-effect studies, apply crossover designs with washout periods ≥7 days, analyzing data via ANOVA with Tukey’s post hoc test .
Q. Basic: How to validate the stability of this compound in long-term storage for longitudinal studies?
Methodological Answer:
- Store aliquots at -80°C in amber vials under argon. Test stability at 0, 6, 12, and 24 months using LC-MS/MS .
- Assess degradation products via high-resolution ion mobility spectrometry (HRIMS) and compare to stress-testing results (e.g., heat, light exposure) .
- Document lot-specific stability in electronic lab notebooks with blockchain timestamps for auditability .
Q. Advanced: How can researchers align this compound studies with evolving regulatory requirements for deuterated drugs?
Methodological Answer:
- Monitor FDA/EMA guidance updates on deuterium substitution, focusing on chemistry, manufacturing, and controls (CMC) .
- For preclinical packages, include deuterium retention studies using mass spectrometry and toxicokinetic assessments of deuterium-related metabolites .
- Engage in pre-IND meetings to align protocols with agency expectations for isotopic effect documentation .
Properties
IUPAC Name |
6-chloro-5-(2,3-dichlorophenoxy)-2-(trideuteriomethylsulfanyl)-1H-benzimidazole | |
---|---|---|
Details | Computed by Lexichem TK 2.7.0 (PubChem release 2021.05.07) | |
Source | PubChem | |
URL | https://pubchem.ncbi.nlm.nih.gov | |
Description | Data deposited in or computed by PubChem | |
InChI |
InChI=1S/C14H9Cl3N2OS/c1-21-14-18-9-5-8(16)12(6-10(9)19-14)20-11-4-2-3-7(15)13(11)17/h2-6H,1H3,(H,18,19)/i1D3 | |
Details | Computed by InChI 1.0.6 (PubChem release 2021.05.07) | |
Source | PubChem | |
URL | https://pubchem.ncbi.nlm.nih.gov | |
Description | Data deposited in or computed by PubChem | |
InChI Key |
NQPDXQQQCQDHHW-FIBGUPNXSA-N | |
Details | Computed by InChI 1.0.6 (PubChem release 2021.05.07) | |
Source | PubChem | |
URL | https://pubchem.ncbi.nlm.nih.gov | |
Description | Data deposited in or computed by PubChem | |
Canonical SMILES |
CSC1=NC2=CC(=C(C=C2N1)Cl)OC3=C(C(=CC=C3)Cl)Cl | |
Details | Computed by OEChem 2.3.0 (PubChem release 2021.05.07) | |
Source | PubChem | |
URL | https://pubchem.ncbi.nlm.nih.gov | |
Description | Data deposited in or computed by PubChem | |
Isomeric SMILES |
[2H]C([2H])([2H])SC1=NC2=CC(=C(C=C2N1)Cl)OC3=C(C(=CC=C3)Cl)Cl | |
Details | Computed by OEChem 2.3.0 (PubChem release 2021.05.07) | |
Source | PubChem | |
URL | https://pubchem.ncbi.nlm.nih.gov | |
Description | Data deposited in or computed by PubChem | |
Molecular Formula |
C14H9Cl3N2OS | |
Details | Computed by PubChem 2.1 (PubChem release 2021.05.07) | |
Source | PubChem | |
URL | https://pubchem.ncbi.nlm.nih.gov | |
Description | Data deposited in or computed by PubChem | |
DSSTOX Substance ID |
DTXSID101043058 | |
Record name | Triclabendazole-(methyl-d3) | |
Source | EPA DSSTox | |
URL | https://comptox.epa.gov/dashboard/DTXSID101043058 | |
Description | DSSTox provides a high quality public chemistry resource for supporting improved predictive toxicology. | |
Molecular Weight |
362.7 g/mol | |
Details | Computed by PubChem 2.1 (PubChem release 2021.05.07) | |
Source | PubChem | |
URL | https://pubchem.ncbi.nlm.nih.gov | |
Description | Data deposited in or computed by PubChem | |
CAS No. |
1353867-93-2 | |
Record name | Triclabendazole-(methyl-d3) | |
Source | EPA DSSTox | |
URL | https://comptox.epa.gov/dashboard/DTXSID101043058 | |
Description | DSSTox provides a high quality public chemistry resource for supporting improved predictive toxicology. | |
Retrosynthesis Analysis
AI-Powered Synthesis Planning: Our tool employs the Template_relevance Pistachio, Template_relevance Bkms_metabolic, Template_relevance Pistachio_ringbreaker, Template_relevance Reaxys, Template_relevance Reaxys_biocatalysis model, leveraging a vast database of chemical reactions to predict feasible synthetic routes.
One-Step Synthesis Focus: Specifically designed for one-step synthesis, it provides concise and direct routes for your target compounds, streamlining the synthesis process.
Accurate Predictions: Utilizing the extensive PISTACHIO, BKMS_METABOLIC, PISTACHIO_RINGBREAKER, REAXYS, REAXYS_BIOCATALYSIS database, our tool offers high-accuracy predictions, reflecting the latest in chemical research and data.
Strategy Settings
Precursor scoring | Relevance Heuristic |
---|---|
Min. plausibility | 0.01 |
Model | Template_relevance |
Template Set | Pistachio/Bkms_metabolic/Pistachio_ringbreaker/Reaxys/Reaxys_biocatalysis |
Top-N result to add to graph | 6 |
Feasible Synthetic Routes
Disclaimer and Information on In-Vitro Research Products
Please be aware that all articles and product information presented on BenchChem are intended solely for informational purposes. The products available for purchase on BenchChem are specifically designed for in-vitro studies, which are conducted outside of living organisms. In-vitro studies, derived from the Latin term "in glass," involve experiments performed in controlled laboratory settings using cells or tissues. It is important to note that these products are not categorized as medicines or drugs, and they have not received approval from the FDA for the prevention, treatment, or cure of any medical condition, ailment, or disease. We must emphasize that any form of bodily introduction of these products into humans or animals is strictly prohibited by law. It is essential to adhere to these guidelines to ensure compliance with legal and ethical standards in research and experimentation.