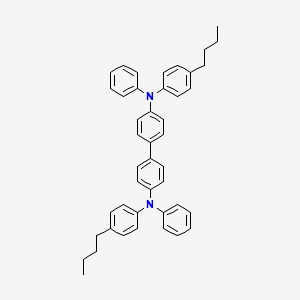
N,N'-Bis(4-butylphenyl)-N,N'-diphenylbenzidine
Overview
Description
N,N’-Bis(4-butylphenyl)-N,N’-diphenylbenzidine, also known as poly-TPD, is a conjugated polymer . It is known for its ability to form thin films of high quality with excellent hole transport, i.e., monopolar electrical conductivity . It has been used in various optoelectronic devices due to its high fluorescence quantum yield and electroluminescence efficiency .
Scientific Research Applications
Charge Carrier Mobility in Polymer Composites
Polymer composites based on poly-TPD with PCBM and copper(II) pyropheophorbide derivative (Cu-PP) were developed . In thin films of the poly-TPD and Cu-PP composites, the charge carrier mobility was investigated for the first time . The electron and hole mobilities in the ternary poly-TPD:PCBM:Cu-PP composite are the most balanced compared to binary composites . The photoconductivity is enhanced due to the sensitization by Cu-PP in blue and red spectral ranges . These new composites are promising for use in the development of photodetectors .
Use in Organic Electronics
Poly-TPD is important in materials design, particularly those related to organic electronics . Its electronic properties can be controlled during both the synthesis and the creation of solid-state materials . Poly-TPD is known for its ability to form thin films of high quality with excellent hole transport, i.e., monopolar electrical conductivity .
Use in Thin Film Devices
Poly-TPD is applicable as a hole transport layer (HTL) in thin film devices due to its highest occupied molecular orbital (HOMO) energy level providing the hole transport matches well with HOMO levels of hole transport polymers and organic photoconductors .
White Light Emission
Poly-TPD can be used to achieve white organic light-emitting diodes (WOLEDs) with a single emitting layer . Monomer emission, excimer, and electromer emission are all observed from poly-TPD, and combining them results in white light emission .
Mechanism of Action
Target of Action
N,N’-Bis(4-butylphenyl)-N,N’-diphenylbenzidine, also known as poly-TPD, is primarily used in the field of photovoltaics, particularly in perovskite solar cells . Its primary target is the hole transport layer (HTL) of these cells .
Mode of Action
Poly-TPD interacts with its target by forming thin films of high quality with excellent hole transport . This is due to its highest occupied molecular orbital (HOMO) energy level providing the hole transport matches well with HOMO levels of hole transport polymers and organic photoconductors .
Biochemical Pathways
In the context of photovoltaics, the biochemical pathways are replaced by electronic pathways. Poly-TPD affects the charge carrier mobility in the HTL of perovskite solar cells . In composites with PCBM and copper (II) pyropheophorbide derivative (Cu-PP), the electron and hole mobilities are balanced, enhancing the photoconductivity due to the sensitization by Cu-PP in blue and red spectral ranges .
Pharmacokinetics
While pharmacokinetics typically refers to the ADME (Absorption, Distribution, Metabolism, Excretion) properties of a drug, in the case of poly-TPD, it’s more relevant to discuss its electronic properties. The hole mobility in thin polymer layers of poly-TPD was reported in the order of (1 ÷ 2) × 10 −3 cm 2 V −1 s −1 .
Result of Action
The introduction of poly-TPD at the perovskite and hole transporting layer interface leads to a significant suppression of interfacial recombination and enhancement of the cell power conversion efficiency . This results in perovskite solar cells with stabilized efficiency exceeding 21% with negligible hysteresis .
Action Environment
Environmental factors such as humidity, heat, and light can influence the action, efficacy, and stability of poly-TPD . For instance, ultra-hydrophobic poly-TPD passivant considerably alleviates moisture penetration, showing ≈91% retention of initial efficiencies after 300 h storage at high relative humidity of 80% .
Future Directions
N,N’-Bis(4-butylphenyl)-N,N’-diphenylbenzidine (poly-TPD) has shown promise in the field of optoelectronics, particularly in the development of photodetectors . The successful energy transfer demonstrated in studies paves the way for exciting future directions in the field of poly-TPD hybrid materials for advanced optoelectronic applications .
properties
IUPAC Name |
4-butyl-N-[4-[4-(N-(4-butylphenyl)anilino)phenyl]phenyl]-N-phenylaniline | |
---|---|---|
Details | Computed by Lexichem TK 2.7.0 (PubChem release 2021.05.07) | |
Source | PubChem | |
URL | https://pubchem.ncbi.nlm.nih.gov | |
Description | Data deposited in or computed by PubChem | |
InChI |
InChI=1S/C44H44N2/c1-3-5-13-35-19-27-41(28-20-35)45(39-15-9-7-10-16-39)43-31-23-37(24-32-43)38-25-33-44(34-26-38)46(40-17-11-8-12-18-40)42-29-21-36(22-30-42)14-6-4-2/h7-12,15-34H,3-6,13-14H2,1-2H3 | |
Details | Computed by InChI 1.0.6 (PubChem release 2021.05.07) | |
Source | PubChem | |
URL | https://pubchem.ncbi.nlm.nih.gov | |
Description | Data deposited in or computed by PubChem | |
InChI Key |
GYPAGHMQEIUKAO-UHFFFAOYSA-N | |
Details | Computed by InChI 1.0.6 (PubChem release 2021.05.07) | |
Source | PubChem | |
URL | https://pubchem.ncbi.nlm.nih.gov | |
Description | Data deposited in or computed by PubChem | |
Canonical SMILES |
CCCCC1=CC=C(C=C1)N(C2=CC=CC=C2)C3=CC=C(C=C3)C4=CC=C(C=C4)N(C5=CC=CC=C5)C6=CC=C(C=C6)CCCC | |
Details | Computed by OEChem 2.3.0 (PubChem release 2021.05.07) | |
Source | PubChem | |
URL | https://pubchem.ncbi.nlm.nih.gov | |
Description | Data deposited in or computed by PubChem | |
Molecular Formula |
C44H44N2 | |
Details | Computed by PubChem 2.1 (PubChem release 2021.05.07) | |
Source | PubChem | |
URL | https://pubchem.ncbi.nlm.nih.gov | |
Description | Data deposited in or computed by PubChem | |
Molecular Weight |
600.8 g/mol | |
Details | Computed by PubChem 2.1 (PubChem release 2021.05.07) | |
Source | PubChem | |
URL | https://pubchem.ncbi.nlm.nih.gov | |
Description | Data deposited in or computed by PubChem | |
Product Name |
N,N'-Bis(4-butylphenyl)-N,N'-diphenylbenzidine |
Synthesis routes and methods
Procedure details
Retrosynthesis Analysis
AI-Powered Synthesis Planning: Our tool employs the Template_relevance Pistachio, Template_relevance Bkms_metabolic, Template_relevance Pistachio_ringbreaker, Template_relevance Reaxys, Template_relevance Reaxys_biocatalysis model, leveraging a vast database of chemical reactions to predict feasible synthetic routes.
One-Step Synthesis Focus: Specifically designed for one-step synthesis, it provides concise and direct routes for your target compounds, streamlining the synthesis process.
Accurate Predictions: Utilizing the extensive PISTACHIO, BKMS_METABOLIC, PISTACHIO_RINGBREAKER, REAXYS, REAXYS_BIOCATALYSIS database, our tool offers high-accuracy predictions, reflecting the latest in chemical research and data.
Strategy Settings
Precursor scoring | Relevance Heuristic |
---|---|
Min. plausibility | 0.01 |
Model | Template_relevance |
Template Set | Pistachio/Bkms_metabolic/Pistachio_ringbreaker/Reaxys/Reaxys_biocatalysis |
Top-N result to add to graph | 6 |
Feasible Synthetic Routes
Disclaimer and Information on In-Vitro Research Products
Please be aware that all articles and product information presented on BenchChem are intended solely for informational purposes. The products available for purchase on BenchChem are specifically designed for in-vitro studies, which are conducted outside of living organisms. In-vitro studies, derived from the Latin term "in glass," involve experiments performed in controlled laboratory settings using cells or tissues. It is important to note that these products are not categorized as medicines or drugs, and they have not received approval from the FDA for the prevention, treatment, or cure of any medical condition, ailment, or disease. We must emphasize that any form of bodily introduction of these products into humans or animals is strictly prohibited by law. It is essential to adhere to these guidelines to ensure compliance with legal and ethical standards in research and experimentation.