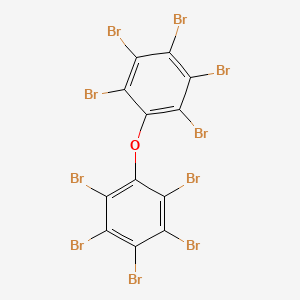
Decabromodiphenyl oxide
Overview
Description
Decabromodiphenyl oxide is a white to off-white powder with a chemical odor. (NTP, 1992)
Decabromodiphenyl ether is a polybromodiphenyl ether that is diphenyl ether in which all of the hydrogens have been replaced by bromines. It has a role as a neurotoxin.
Mechanism of Action
Target of Action
Decabromodiphenyl ether (decaBDE), a member of the Poly Brominated Diphenyl Ether family (PBDEs), is a highly persistent flame retardant . It is known to accumulate in lipid-rich tissues and primarily targets the liver due to its high lipophilicity . Additionally, it has been found to bind with thyroid hormone receptors (TRs), thyroid binding globulin (TBG), transthyretin (TTR), and related enzymes .
Mode of Action
DecaBDE is initially absorbed by wall teichoic acid and N-acetylglucosamine side chains in peptidoglycan . It is then transported and debrominated through three pathways, giving tri-, hepta-, octa-, and nona-bromodiphenyl ethers . The C–C bond energies decrease as the number of bromine atoms on the diphenyl decreases .
Biochemical Pathways
DecaBDE undergoes phototransformation in various media, such as n-hexane, MeOH:H2O, and simulated seawater . The main mechanism for decaBDE phototransformation in n-hexane is debromination with cleavage of C–Br bonds as the rate-limiting step . In MeOH:H2O, the rate-limiting steps are debromination and hydroxylation with cleavage of C–Br bonds . In simulated seawater, the mechanisms include debromination, hydroxylation, and chlorination .
Pharmacokinetics
DecaBDE is known for its environmental persistence and potential health risks . It is highly capable of bioaccumulation and food web biomagnification . Little is known about the kinetics of pbdes, especially the highly brominated bde congeners .
Result of Action
PBDEs inhibit protein expression or accelerate protein degradation and increase membrane permeability . They also increase the release of Cl−, Na+, NH4+, arabinose, proteins, acetic acid, and oxalic acid . Pbdes increase the amounts of k+, mg2+, po43−, so42−, and no3− assimilated .
Action Environment
DecaBDE is extremely persistent and capable of long-distance transport . It is widely used in various industries such as electronics, furniture, plastics, and textiles . The sources of decaBDE environmental pollution are its manufacturing and flame-retardant plastic modification plants . The transformation of decaBDE is influenced by environmental factors such as sunlight and the medium in which it is present .
Biochemical Analysis
Biochemical Properties
Decabromodiphenyl ether is initially absorbed by wall teichoic acid and N-acetylglucosamine side chains in peptidoglycan, and then it is transported and debrominated through three pathways, giving tri-, hepta-, octa-, and nona-bromodiphenyl ethers . The C–C bond energies decrease as the number of bromine atoms on the diphenyl decreases . It has been found to interact with thyroid hormone receptors (TRs), thyroid binding globulin (TBG), transthyretin (TTR), and related enzymes due to its chemical structures that are similar to those of T3 and T4 .
Cellular Effects
Decabromodiphenyl ether has been reported to harm reproductive health . It inhibits protein expression or accelerates protein degradation and increases membrane permeability . It also influences cell function by increasing the amounts of K+, Mg2+, PO43−, SO42−, and NO3− assimilated .
Molecular Mechanism
Decabromodiphenyl ether undergoes debromination in the presence of microbe and zero-valent iron . It is also involved in phototransformation mechanisms in various media . The compound-specific stable isotope analysis was first applied to investigate its phototransformation .
Temporal Effects in Laboratory Settings
Decabromodiphenyl ether is extremely persistent and capable of long-distance transport . Soil samples treated with it remained non-degradable even after 180 days in the absence of light .
Dosage Effects in Animal Models
Based on animal model studies, Decabromodiphenyl ether produces direct and indirect toxic effects on the thyroid gland . Higher doses of it may cause the reduction of thyroglobulin (TG) and thyroid peroxidase (TPO) by the atrophy of the rough endoplasmic reticulum .
Metabolic Pathways
Decabromodiphenyl ether is transported and debrominated through three pathways, giving tri-, hepta-, octa-, and nona-bromodiphenyl ethers . Multiple dehalogenases were found in the genome of Rhodococcus ruber TAW-CT127; these may be involved in the production of lower-brominated diphenyl ethers .
Transport and Distribution
Decabromodiphenyl ether is highly capable of bioaccumulation and food web bio magnification . It is transported and debrominated through three pathways, giving tri-, hepta-, octa-, and nona-bromodiphenyl ethers .
Subcellular Localization
It is known to interact with various cellular components and influence their functions
Properties
CAS No. |
562099-68-7 |
---|---|
Molecular Formula |
C12Br10O |
Molecular Weight |
971.1 g/mol |
IUPAC Name |
1,2,3,4,5-pentabromo-6-(2,3,4,5,6-pentabromo(1,2,3,4,5,6-13C6)cyclohexa-1,3,5-trien-1-yl)oxy(1,2,3,4,5,6-13C6)cyclohexa-1,3,5-triene |
InChI |
InChI=1S/C12Br10O/c13-1-3(15)7(19)11(8(20)4(1)16)23-12-9(21)5(17)2(14)6(18)10(12)22/i1+1,2+1,3+1,4+1,5+1,6+1,7+1,8+1,9+1,10+1,11+1,12+1 |
InChI Key |
WHHGLZMJPXIBIX-WCGVKTIYSA-N |
Isomeric SMILES |
[13C]1(=[13C]([13C](=[13C]([13C](=[13C]1Br)Br)Br)Br)Br)O[13C]2=[13C]([13C](=[13C]([13C](=[13C]2Br)Br)Br)Br)Br |
impurities |
The commercially available grades are >98% decabromodiphenyl oxide with the remainder being the nonabromo species. |
SMILES |
C1(=C(C(=C(C(=C1Br)Br)Br)Br)Br)OC2=C(C(=C(C(=C2Br)Br)Br)Br)Br |
Canonical SMILES |
C1(=C(C(=C(C(=C1Br)Br)Br)Br)Br)OC2=C(C(=C(C(=C2Br)Br)Br)Br)Br |
boiling_point |
530.0 °C Decomposes at 425 °C |
Color/Form |
Yellow prisms from toluene White powde |
density |
3 (NTP, 1992) 3.4 Relative density (water = 1): 3.0 |
melting_point |
569.1 to 576.5 °F (NTP, 1992) 295.0 °C 305 °C 300-310 °C |
physical_description |
Decabromodiphenyl oxide is a white to off-white powder with a chemical odor. (NTP, 1992) White solid; [HSDB] Faintly beige powder; [MSDSonline] WHITE CRYSTALLINE POWDER. |
solubility |
less than 1 mg/mL at 68° F (NTP, 1992) In water, <1.0X10-4 mg/L at 25 °C /OECD Guideline 105 (Water Solubility)/ Solubility (wt%): 0.05 acetone; 0.1 toluene; 0.48 benzene; 0.42 methylene bromide; 0.87 xylene Solubility in water: none |
vapor_pressure |
less than 1 mm Hg at 68 °F (NTP, 1992) 6.96X10-11 mm Hg at 25 °C /extrapolated/ Vapor pressure, Pa at 21 °C: (negligible) |
Origin of Product |
United States |
Retrosynthesis Analysis
AI-Powered Synthesis Planning: Our tool employs the Template_relevance Pistachio, Template_relevance Bkms_metabolic, Template_relevance Pistachio_ringbreaker, Template_relevance Reaxys, Template_relevance Reaxys_biocatalysis model, leveraging a vast database of chemical reactions to predict feasible synthetic routes.
One-Step Synthesis Focus: Specifically designed for one-step synthesis, it provides concise and direct routes for your target compounds, streamlining the synthesis process.
Accurate Predictions: Utilizing the extensive PISTACHIO, BKMS_METABOLIC, PISTACHIO_RINGBREAKER, REAXYS, REAXYS_BIOCATALYSIS database, our tool offers high-accuracy predictions, reflecting the latest in chemical research and data.
Strategy Settings
Precursor scoring | Relevance Heuristic |
---|---|
Min. plausibility | 0.01 |
Model | Template_relevance |
Template Set | Pistachio/Bkms_metabolic/Pistachio_ringbreaker/Reaxys/Reaxys_biocatalysis |
Top-N result to add to graph | 6 |
Feasible Synthetic Routes
Q1: What is the molecular formula and weight of Decabromodiphenyl ether?
A1: Decabromodiphenyl ether is represented by the molecular formula C12Br10O and has a molecular weight of 959.2 g/mol.
Q2: What are the key spectroscopic characteristics of Decabromodiphenyl ether?
A2: While specific spectroscopic data may vary depending on the study and analytical techniques employed, studies commonly utilize techniques like gas chromatography-mass spectrometry (GC-MS) [, ] and Fourier transform-infrared spectrometry (FT-IR) [] to identify and quantify DBDPE and its degradation products.
Q3: In which materials and applications is Decabromodiphenyl ether commonly used?
A3: DBDPE is predominantly incorporated into polymers like high-impact polystyrene (HIPS) [] and epoxy resins [, ] to impart flame-retardant properties. These materials find extensive use in electronic casings, textiles, and construction materials.
Q4: How does the presence of Decabromodiphenyl ether affect the thermal properties of polymers?
A4: Research indicates that DBDPE, in conjunction with antimony trioxide, can significantly influence the thermal degradation behavior of polymers like HIPS []. This synergistic effect enhances flame retardancy by altering the decomposition pathway and promoting char formation.
Q5: Are there any known compatibility issues with Decabromodiphenyl ether in specific polymer matrices?
A5: Research suggests that while generally compatible, the incorporation of DBDPE can impact the mechanical properties of certain polymers []. For instance, the addition of DBDPE, especially in combination with antimony trioxide, may decrease the tensile strength of EPDM/PP blends [].
Q6: What are the primary pathways for Decabromodiphenyl ether release into the environment?
A6: DBDPE can enter the environment through various pathways, including manufacturing processes, leaching from products during use, and improper disposal [, ]. Its presence has been documented in diverse environmental compartments, including sediments, water bodies, and air.
Q7: How persistent is Decabromodiphenyl ether in the environment?
A7: DBDPE exhibits considerable persistence in various environmental matrices [, ]. While it can undergo degradation processes like photolysis and biodegradation, these processes tend to be slow, leading to its accumulation in the environment.
Q8: Does Decabromodiphenyl ether undergo any significant transformations in the environment?
A8: Yes, research has shown that DBDPE can be subject to debromination, a process where bromine atoms are removed from the molecule [, , , , , ]. This process often results in the formation of lower brominated diphenyl ethers, some of which may exhibit greater toxicity than the parent compound.
Q9: What are the known toxicological effects of Decabromodiphenyl ether on aquatic organisms?
A9: Studies using Daphnia magna and Microcystis flos-aquae have revealed that DBDPE and its degradation products can exert toxic effects on aquatic organisms []. The intermediate products formed during its degradation, particularly via reductive pathways, were found to be more toxic than DBDPE itself, highlighting the importance of understanding its transformation pathways.
Q10: What is the potential for Decabromodiphenyl ether bioaccumulation in food webs?
A10: Research has documented the bioaccumulation of DBDPE in both terrestrial and aquatic food webs [, , ]. While its bioaccumulation potential might be lower than that of lower brominated PBDEs, it can still pose risks to organisms at higher trophic levels, including humans.
Q11: What is being done to mitigate the environmental risks associated with Decabromodiphenyl ether?
A11: Several approaches are being explored to minimize the environmental impact of DBDPE, including the development of alternative flame retardants [], improved recycling and waste management strategies [, ], and bioremediation techniques utilizing microorganisms capable of degrading DBDPE [, ].
Q12: What are the main routes of human exposure to Decabromodiphenyl ether?
A12: Humans are primarily exposed to DBDPE through dietary intake, inhalation of dust contaminated with DBDPE leached from products, and dermal contact with treated materials [, , ].
Q13: Have there been studies on the potential health effects of Decabromodiphenyl ether in humans?
A13: While controlled clinical trials investigating DBDPE's direct effects on humans are limited, numerous studies have explored its potential adverse effects using in vitro cell-based assays and in vivo animal models [, , , , ].
Q14: What are the potential endocrine-disrupting effects of Decabromodiphenyl ether?
A14: Research suggests that DBDPE might interfere with thyroid hormone homeostasis [, , ]. This is particularly concerning given the critical role of thyroid hormones in growth, development, and metabolism.
Q15: Has Decabromodiphenyl ether been linked to any carcinogenic effects?
A15: Studies investigating the potential carcinogenicity of DBDPE have yielded mixed results. While some animal studies have shown limited evidence of carcinogenicity [], others suggest potential links to specific types of cancer, warranting further investigation [, ].
Q16: What are the potential neurodevelopmental effects of Decabromodiphenyl ether exposure?
A16: Animal studies have raised concerns regarding the potential neurodevelopmental toxicity of DBDPE, particularly following prenatal and early life exposure [, ]. These studies highlight the need to assess the vulnerability of developing organisms to DBDPE exposure.
Q17: What analytical methods are employed for detecting and quantifying Decabromodiphenyl ether in environmental samples?
A17: Various analytical techniques are used to detect and measure DBDPE, with gas chromatography coupled with mass spectrometry (GC-MS) being one of the most widely employed methods [, , ]. This method enables the separation and quantification of different PBDE congeners, including DBDPE.
Q18: Are there any specific challenges associated with the analysis of Decabromodiphenyl ether in environmental and biological matrices?
A18: The analysis of DBDPE can be challenging due to its high molecular weight and low volatility [, ]. These properties necessitate careful sample preparation and extraction procedures to ensure accurate and reliable measurements.
Q19: What are the future directions for research on Decabromodiphenyl ether?
A19: Future research should prioritize a more comprehensive understanding of DBDPE's long-term health effects, its degradation pathways in various environmental compartments, and the development of sustainable and effective remediation strategies [, , ].
Disclaimer and Information on In-Vitro Research Products
Please be aware that all articles and product information presented on BenchChem are intended solely for informational purposes. The products available for purchase on BenchChem are specifically designed for in-vitro studies, which are conducted outside of living organisms. In-vitro studies, derived from the Latin term "in glass," involve experiments performed in controlled laboratory settings using cells or tissues. It is important to note that these products are not categorized as medicines or drugs, and they have not received approval from the FDA for the prevention, treatment, or cure of any medical condition, ailment, or disease. We must emphasize that any form of bodily introduction of these products into humans or animals is strictly prohibited by law. It is essential to adhere to these guidelines to ensure compliance with legal and ethical standards in research and experimentation.