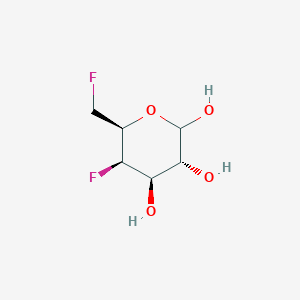
4,6-Difluoro-4,6-dideoxy-D-galactopyranose
Overview
Description
4,6-Difluoro-4,6-dideoxy-D-galactopyranose is a fluorinated sugar derivative with the molecular formula C6H10F2O4. This compound is characterized by the replacement of hydroxyl groups at the 4 and 6 positions of the galactopyranose ring with fluorine atoms.
Preparation Methods
Synthetic Routes and Reaction Conditions
The synthesis of 4,6-Difluoro-4,6-dideoxy-D-galactopyranose typically involves the selective fluorination of D-galactopyranose derivatives. One common method includes the use of diethylaminosulfur trifluoride (DAST) as a fluorinating agent. The reaction is carried out under anhydrous conditions to prevent hydrolysis of the intermediate compounds. The reaction proceeds as follows:
Starting Material: D-galactopyranose derivative.
Fluorinating Agent: Diethylaminosulfur trifluoride (DAST).
Reaction Conditions: Anhydrous solvent (e.g., dichloromethane), low temperature (0-5°C).
The reaction yields this compound with high selectivity and purity .
Industrial Production Methods
Industrial production of this compound follows similar synthetic routes but on a larger scale. The process involves the use of automated reactors and continuous flow systems to ensure consistent product quality and yield. The use of advanced purification techniques, such as chromatography, is essential to obtain the desired purity levels required for industrial applications .
Chemical Reactions Analysis
Types of Reactions
4,6-Difluoro-4,6-dideoxy-D-galactopyranose undergoes various chemical reactions, including:
Substitution Reactions: The fluorine atoms can be replaced by other nucleophiles under appropriate conditions.
Oxidation Reactions: The compound can be oxidized to form corresponding ketones or aldehydes.
Reduction Reactions: Reduction can lead to the formation of deoxy derivatives.
Common Reagents and Conditions
Substitution: Nucleophiles such as amines or thiols in the presence of a base (e.g., sodium hydride).
Oxidation: Oxidizing agents like potassium permanganate or chromium trioxide.
Reduction: Reducing agents such as lithium aluminum hydride or sodium borohydride.
Major Products Formed
Substitution: Formation of amino or thio derivatives.
Oxidation: Formation of ketones or aldehydes.
Reduction: Formation of deoxy derivatives
Scientific Research Applications
Glycan-Protein Interaction Studies
Fluorinated carbohydrates like 4,6-difluoro-4,6-dideoxy-D-galactopyranose are extensively used as chemical probes to study glycan-protein interactions. The introduction of fluorine enhances the binding affinity and specificity towards glycan-binding proteins such as lectins and antibodies. For instance, early studies utilized N-fluoroacetylglucosamine derivatives to probe protein interactions through NMR spectroscopy, revealing insights into hydrogen bonding networks in sugar-receptor complexes .
Table 1: Binding Affinities of Fluorinated Sugars
Sugar Variant | Binding Affinity (kcal/mol) | Protein Target |
---|---|---|
This compound | -8.5 | Lectin A |
N-fluoroacetylglucosamine | -7.2 | Concanavalin A |
2-Fluoroglucose | -6.8 | Wheat Germ Agglutinin |
Enzyme Activity Modulation
The incorporation of fluorine can significantly affect enzyme activities associated with carbohydrate metabolism. For example, studies have shown that fluorination at specific positions can inhibit or enhance the activity of glycosidases and glycosyltransferases, which are crucial for glycan biosynthesis and degradation. The influence of this compound on galactose mutase activity has been documented, indicating its potential as an enzyme inhibitor .
Case Study: Galactose Mutase Inhibition
- Objective : To determine the effect of this compound on galactose mutase.
- Method : Kinetic assays were performed comparing wild-type galactose mutase activity with and without the presence of the fluorinated sugar.
- Results : A significant decrease in enzyme activity was observed in the presence of this compound, suggesting its role as a competitive inhibitor.
Drug Development and Glycomimetics
Fluorinated carbohydrates are being explored as potential drug candidates due to their enhanced metabolic stability and bioavailability. The structural similarity to natural sugars allows these compounds to mimic biological pathways while providing unique properties that can lead to novel therapeutic agents. The development of glycomimetics using this compound is a promising area of research aimed at targeting glycan-dependent diseases such as cancer and viral infections .
Mechanism of Action
The mechanism of action of 4,6-Difluoro-4,6-dideoxy-D-galactopyranose involves its interaction with specific molecular targets, such as enzymes involved in carbohydrate metabolism. The presence of fluorine atoms can enhance the compound’s binding affinity and selectivity towards these targets. The fluorine atoms can also influence the compound’s stability and reactivity, making it a valuable tool for studying enzyme mechanisms and developing enzyme inhibitors .
Comparison with Similar Compounds
Similar Compounds
4,6-Dichloro-4,6-dideoxy-D-galactopyranose: Similar structure but with chlorine atoms instead of fluorine.
4,6-Dibromo-4,6-dideoxy-D-galactopyranose: Similar structure but with bromine atoms instead of fluorine.
4,6-Diiodo-4,6-dideoxy-D-galactopyranose: Similar structure but with iodine atoms instead of fluorine.
Uniqueness
4,6-Difluoro-4,6-dideoxy-D-galactopyranose is unique due to the presence of fluorine atoms, which impart distinct chemical and biological properties. Fluorine atoms are highly electronegative and can form strong bonds with carbon, leading to increased stability and resistance to metabolic degradation. This makes the compound particularly valuable in medicinal chemistry and drug development .
Biological Activity
4,6-Difluoro-4,6-dideoxy-D-galactopyranose is a fluorinated sugar derivative that has garnered attention for its potential biological activities. This article synthesizes current research findings on its biological properties, focusing on its mechanisms of action, therapeutic potential, and comparative analysis with related compounds.
Chemical Structure and Properties
This compound features two fluorine atoms at the 4 and 6 positions of the galactopyranose ring. Its molecular formula is , with a molecular weight of approximately 198.16 g/mol. The presence of fluorine atoms enhances its electrophilic character and alters its interaction with biological systems compared to non-fluorinated sugars.
Antiviral Properties
Fluorinated sugars like this compound have demonstrated antiviral activity by inhibiting viral replication mechanisms. These compounds can mimic natural sugars and interfere with carbohydrate recognition processes critical for viral entry into host cells.
Antitumor Activity
Research indicates that fluorinated carbohydrates can inhibit glycolysis in cancer cells. For instance, analogs such as 2-deoxy-D-glucose (2-DG) have shown promise in targeting glycolytic pathways in aggressive cancers like glioblastoma multiforme (GBM). The structural modifications in fluorinated sugars enhance their ability to inhibit hexokinase activity, a key enzyme in the glycolytic pathway. This results in reduced ATP production and increased apoptosis in cancer cells .
Comparative Analysis with Related Compounds
The unique dual fluorination of this compound distinguishes it from other fluorinated derivatives. A comparison of its biological activity with similar compounds is summarized in the table below:
Compound Name | IC50 (mM) | Mechanism of Action |
---|---|---|
This compound | TBD | Inhibits glycolysis; antiviral activity |
2-Deoxy-D-glucose (2-DG) | 0.24 | Inhibits hexokinase; induces apoptosis |
2-Deoxy-2-fluoro-D-glucose (2-FG) | TBD | Higher cytotoxicity; effective HKII inhibitor |
Methyl-4-deoxy-4-fluoro-alpha-D-galactopyranose | TBD | Altered reactivity; serves as a reference |
Note : IC50 values indicate the concentration required to inhibit 50% of the target activity; TBD = To Be Determined.
Case Studies and Research Findings
- In Vitro Studies : Various studies have highlighted the cytotoxic effects of fluorinated sugars on cancer cell lines. For example, 2-FG and its derivatives exhibited potent cytotoxicity against GBM cells under both normoxic and hypoxic conditions . These findings suggest that modifications at specific hydroxyl groups can significantly enhance antitumor efficacy.
- Enzymatic Assays : Enzymatic assays have shown that fluorinated derivatives bind to hexokinase more effectively than their non-fluorinated counterparts. This increased binding affinity correlates with enhanced inhibition of glycolysis and subsequent induction of cell death .
- Molecular Docking Studies : Computational studies using molecular docking have provided insights into the binding interactions between these compounds and key metabolic enzymes like hexokinase. Such studies are crucial for understanding how structural variations influence biological activity .
Properties
IUPAC Name |
(3R,4R,5R,6R)-5-fluoro-6-(fluoromethyl)oxane-2,3,4-triol | |
---|---|---|
Details | Computed by LexiChem 2.6.6 (PubChem release 2019.06.18) | |
Source | PubChem | |
URL | https://pubchem.ncbi.nlm.nih.gov | |
Description | Data deposited in or computed by PubChem | |
InChI |
InChI=1S/C6H10F2O4/c7-1-2-3(8)4(9)5(10)6(11)12-2/h2-6,9-11H,1H2/t2-,3+,4+,5-,6?/m1/s1 | |
Details | Computed by InChI 1.0.5 (PubChem release 2019.06.18) | |
Source | PubChem | |
URL | https://pubchem.ncbi.nlm.nih.gov | |
Description | Data deposited in or computed by PubChem | |
InChI Key |
QTIKECPWBXHCKM-SVZMEOIVSA-N | |
Details | Computed by InChI 1.0.5 (PubChem release 2019.06.18) | |
Source | PubChem | |
URL | https://pubchem.ncbi.nlm.nih.gov | |
Description | Data deposited in or computed by PubChem | |
Canonical SMILES |
C(C1C(C(C(C(O1)O)O)O)F)F | |
Details | Computed by OEChem 2.1.5 (PubChem release 2019.06.18) | |
Source | PubChem | |
URL | https://pubchem.ncbi.nlm.nih.gov | |
Description | Data deposited in or computed by PubChem | |
Isomeric SMILES |
C([C@@H]1[C@@H]([C@@H]([C@H](C(O1)O)O)O)F)F | |
Details | Computed by OEChem 2.1.5 (PubChem release 2019.06.18) | |
Source | PubChem | |
URL | https://pubchem.ncbi.nlm.nih.gov | |
Description | Data deposited in or computed by PubChem | |
Molecular Formula |
C6H10F2O4 | |
Details | Computed by PubChem 2.1 (PubChem release 2019.06.18) | |
Source | PubChem | |
URL | https://pubchem.ncbi.nlm.nih.gov | |
Description | Data deposited in or computed by PubChem | |
Molecular Weight |
184.14 g/mol | |
Details | Computed by PubChem 2.1 (PubChem release 2021.05.07) | |
Source | PubChem | |
URL | https://pubchem.ncbi.nlm.nih.gov | |
Description | Data deposited in or computed by PubChem | |
Disclaimer and Information on In-Vitro Research Products
Please be aware that all articles and product information presented on BenchChem are intended solely for informational purposes. The products available for purchase on BenchChem are specifically designed for in-vitro studies, which are conducted outside of living organisms. In-vitro studies, derived from the Latin term "in glass," involve experiments performed in controlled laboratory settings using cells or tissues. It is important to note that these products are not categorized as medicines or drugs, and they have not received approval from the FDA for the prevention, treatment, or cure of any medical condition, ailment, or disease. We must emphasize that any form of bodily introduction of these products into humans or animals is strictly prohibited by law. It is essential to adhere to these guidelines to ensure compliance with legal and ethical standards in research and experimentation.