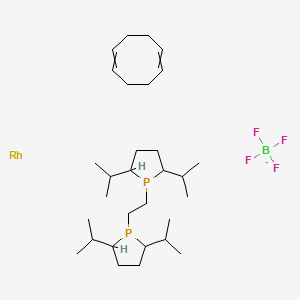
1,2-Bis((2R,5R)-2,5-di-i-propylphospholano)ethane(cyclooctadiene)rhodium(I)
- Click on QUICK INQUIRY to receive a quote from our team of experts.
- With the quality product at a COMPETITIVE price, you can focus more on your research.
Overview
Description
1,2-Bis((2R,5R)-2,5-di-i-propylphospholano)ethane(cyclooctadiene)rhodium(I), also known as (R,R)-COD Rh(I) or Crabtree's catalyst, is a transition metal complex that has been widely used in organic chemistry research. It was first synthesized by James P. Collman and his team in 1975, and since then, it has been extensively studied due to its unique properties and applications in various fields.
Scientific Research Applications
Asymmetric Hydrogenation Catalyst
This compound is used as a catalyst for the asymmetric hydrogenation of N-acetyldehydroamino acid precursors . This process is crucial in the preparation of substituted phenylalanine, an important amino acid in biochemistry .
2. Enantioselective Preparation of Trifluoromethyl Amino Acids It is also used in the enantioselective preparation of trifluoromethyl amino acids . These amino acids are important in medicinal chemistry due to their bioisosteric properties .
Asymmetric Hydrogenation of Acetamidocinnamate
Another application of this compound is in the asymmetric hydrogenation of acetamidocinnamate . This reaction is a key step in the synthesis of various pharmaceutical compounds .
Preparation of Substituted Phenylalanine
This compound is used in the preparation of substituted phenylalanine via asymmetric hydrogenation . Substituted phenylalanine is a crucial building block in the synthesis of various bioactive compounds .
Asymmetric Hydrogenation of Cyclobutyl Enamides
It is also used in the asymmetric hydrogenation of cyclobutyl enamides . This reaction is a key step in the synthesis of cyclobutane-containing amino acids .
6. Preparation of Chiral Cbz-Aminodifluorobutyric Acid Me Ester This compound is used in the asymmetric preparation of chiral Cbz-aminodifluorobutyric acid Me ester and its analogs . These compounds are important in the synthesis of various bioactive compounds .
Mechanism of Action
Target of Action
The primary target of 1,2-Bis((2R,5R)-2,5-di-i-propylphospholano)ethane(cyclooctadiene)rhodium(I) is the substrate in asymmetric hydrogenation reactions . This compound, a chiral ligand, forms a metal complex with rhodium, which acts as a catalyst in these reactions .
Mode of Action
The compound interacts with its target by forming a Duphos-Rhodium metal complex . This complex then catalyzes the asymmetric hydrogenation of the substrate . The interaction results in the formation of a single-enantiomer product .
Biochemical Pathways
The compound affects the asymmetric hydrogenation pathway . The downstream effects include the stereoselective synthesis of various compounds, such as δ-amino acid derivatives, manzacidins A and C, and the tetracyclic core of manzamine A .
Result of Action
The molecular effect of the compound’s action is the conversion of a substrate into a single-enantiomer product . On a cellular level, this could potentially influence the activity of enzymes and other proteins that interact with the product.
Action Environment
Environmental factors such as temperature, pH, and the presence of other compounds can influence the compound’s action, efficacy, and stability. For instance, the compound is air sensitive , indicating that its stability and efficacy might decrease upon exposure to air. Furthermore, the reaction conditions, such as the solvent used and the presence of other catalysts, can also affect the compound’s action .
properties
IUPAC Name |
cycloocta-1,5-diene;1-[2-[2,5-di(propan-2-yl)phospholan-1-yl]ethyl]-2,5-di(propan-2-yl)phospholane;rhodium;tetrafluoroborate |
Source
|
---|---|---|
Source | PubChem | |
URL | https://pubchem.ncbi.nlm.nih.gov | |
Description | Data deposited in or computed by PubChem | |
InChI |
InChI=1S/C22H44P2.C8H12.BF4.Rh/c1-15(2)19-9-10-20(16(3)4)23(19)13-14-24-21(17(5)6)11-12-22(24)18(7)8;1-2-4-6-8-7-5-3-1;2-1(3,4)5;/h15-22H,9-14H2,1-8H3;1-2,7-8H,3-6H2;;/q;;-1; |
Source
|
Source | PubChem | |
URL | https://pubchem.ncbi.nlm.nih.gov | |
Description | Data deposited in or computed by PubChem | |
InChI Key |
NRQFPKPBTSPMQF-UHFFFAOYSA-N |
Source
|
Source | PubChem | |
URL | https://pubchem.ncbi.nlm.nih.gov | |
Description | Data deposited in or computed by PubChem | |
Canonical SMILES |
[B-](F)(F)(F)F.CC(C)C1CCC(P1CCP2C(CCC2C(C)C)C(C)C)C(C)C.C1CC=CCCC=C1.[Rh] |
Source
|
Source | PubChem | |
URL | https://pubchem.ncbi.nlm.nih.gov | |
Description | Data deposited in or computed by PubChem | |
Molecular Formula |
C30H56BF4P2Rh- |
Source
|
Source | PubChem | |
URL | https://pubchem.ncbi.nlm.nih.gov | |
Description | Data deposited in or computed by PubChem | |
Molecular Weight |
668.4 g/mol |
Source
|
Source | PubChem | |
URL | https://pubchem.ncbi.nlm.nih.gov | |
Description | Data deposited in or computed by PubChem | |
Product Name |
1,2-Bis((2R,5R)-2,5-di-i-propylphospholano)ethane(cyclooctadiene)rhodium(I) |
Disclaimer and Information on In-Vitro Research Products
Please be aware that all articles and product information presented on BenchChem are intended solely for informational purposes. The products available for purchase on BenchChem are specifically designed for in-vitro studies, which are conducted outside of living organisms. In-vitro studies, derived from the Latin term "in glass," involve experiments performed in controlled laboratory settings using cells or tissues. It is important to note that these products are not categorized as medicines or drugs, and they have not received approval from the FDA for the prevention, treatment, or cure of any medical condition, ailment, or disease. We must emphasize that any form of bodily introduction of these products into humans or animals is strictly prohibited by law. It is essential to adhere to these guidelines to ensure compliance with legal and ethical standards in research and experimentation.